stm32f103c8t6用pid算法控制五路循迹小车
时间: 2023-09-15 08:17:16 浏览: 36
好的,让我们来讨论如何使用PID算法控制五路循迹小车。
首先,我们需要了解PID控制的基本原理和公式。PID控制器的输入是当前误差值e(t),输出是控制量u(t),控制器的计算公式为:
u(t) = Kp * e(t) + Ki * ∫e(t) dt + Kd * de(t)/dt
其中Kp、Ki和Kd分别代表比例、积分和微分系数,e(t)代表当前误差,de(t)/dt代表误差变化率,∫e(t) dt代表误差的积分项。
接下来,我们需要明确五路循迹小车的控制目标。五路循迹小车的目标是在黑线上行驶,因此我们可以将五路循迹传感器的输出值作为误差e(t),控制小车行驶。
具体实现步骤如下:
1. 初始化PID控制器的参数Kp、Ki、Kd和误差积分项I,将I初始化为0。
2. 读取五路循迹传感器的输出值,计算误差e(t)。
3. 将误差e(t)和误差变化率de(t)/dt传入PID控制器,计算控制量u(t)。
4. 根据控制量u(t)控制小车的速度和方向。例如,如果控制量u(t)为正,小车向左转;如果控制量u(t)为负,小车向右转。
5. 将控制器的输出值作为反馈信号,返回步骤2。
需要注意的是,在实际控制过程中,需要对PID控制器进行调参,以达到最优的控制效果。具体调参方法可以参考经典的Ziegler-Nichols方法或其他自适应控制方法。
相关问题
stm32f103c8t6用pid算法控制五路循迹小车(无需测速,用直流电机控制)
好的,下面是一个简单的基于STM32F103C8T6的PID算法控制五路循迹小车的示例代码:
```c
#include "stm32f10x.h"
// 定义电机控制引脚
#define MOTOR_1_A_Pin GPIO_Pin_0
#define MOTOR_1_B_Pin GPIO_Pin_1
#define MOTOR_2_A_Pin GPIO_Pin_2
#define MOTOR_2_B_Pin GPIO_Pin_3
#define MOTOR_3_A_Pin GPIO_Pin_4
#define MOTOR_3_B_Pin GPIO_Pin_5
#define MOTOR_4_A_Pin GPIO_Pin_6
#define MOTOR_4_B_Pin GPIO_Pin_7
#define MOTOR_5_A_Pin GPIO_Pin_8
#define MOTOR_5_B_Pin GPIO_Pin_9
// 定义循迹传感器引脚
#define SENSOR_1_Pin GPIO_Pin_10
#define SENSOR_2_Pin GPIO_Pin_11
#define SENSOR_3_Pin GPIO_Pin_12
#define SENSOR_4_Pin GPIO_Pin_13
#define SENSOR_5_Pin GPIO_Pin_14
// PID参数
double kp = 0.5;
double ki = 0.1;
double kd = 0.1;
// 循迹传感器阈值
int threshold = 500;
// 当前偏差
int currentError = 0;
// 上一次偏差
int lastError = 0;
// 积分项
double integral = 0;
// 微分项
double derivative = 0;
// 目标速度(PWM占空比)
int targetSpeed = 100;
// 左右电机PWM值
int pwmLeft = 0;
int pwmRight = 0;
// 初始化GPIO
void initGPIO()
{
RCC_APB2PeriphClockCmd(RCC_APB2Periph_GPIOA, ENABLE);
RCC_APB2PeriphClockCmd(RCC_APB2Periph_GPIOB, ENABLE);
GPIO_InitTypeDef GPIO_InitStruct;
GPIO_InitStruct.GPIO_Pin = MOTOR_1_A_Pin | MOTOR_1_B_Pin | MOTOR_2_A_Pin | MOTOR_2_B_Pin | MOTOR_3_A_Pin | MOTOR_3_B_Pin | MOTOR_4_A_Pin | MOTOR_4_B_Pin | MOTOR_5_A_Pin | MOTOR_5_B_Pin;
GPIO_InitStruct.GPIO_Mode = GPIO_Mode_Out_PP;
GPIO_InitStruct.GPIO_Speed = GPIO_Speed_50MHz;
GPIO_Init(GPIOA, &GPIO_InitStruct);
GPIO_InitStruct.GPIO_Pin = SENSOR_1_Pin | SENSOR_2_Pin | SENSOR_3_Pin | SENSOR_4_Pin | SENSOR_5_Pin;
GPIO_InitStruct.GPIO_Mode = GPIO_Mode_AIN;
GPIO_Init(GPIOB, &GPIO_InitStruct);
}
// 读取循迹传感器值
void readSensors(int *sensorValues)
{
sensorValues[0] = ADC_GetConversionValue(ADC1);
sensorValues[1] = ADC_GetConversionValue(ADC2);
sensorValues[2] = ADC_GetConversionValue(ADC3);
sensorValues[3] = ADC_GetConversionValue(ADC4);
sensorValues[4] = ADC_GetConversionValue(ADC5);
}
// 控制电机
void controlMotors(int pwmLeft, int pwmRight)
{
if (pwmLeft > 0)
{
GPIO_SetBits(GPIOA, MOTOR_1_A_Pin);
GPIO_ResetBits(GPIOA, MOTOR_1_B_Pin);
TIM_SetCompare1(TIM1, pwmLeft);
}
else if (pwmLeft < 0)
{
GPIO_ResetBits(GPIOA, MOTOR_1_A_Pin);
GPIO_SetBits(GPIOA, MOTOR_1_B_Pin);
TIM_SetCompare1(TIM1, -pwmLeft);
}
else
{
GPIO_ResetBits(GPIOA, MOTOR_1_A_Pin);
GPIO_ResetBits(GPIOA, MOTOR_1_B_Pin);
TIM_SetCompare1(TIM1, 0);
}
if (pwmRight > 0)
{
GPIO_SetBits(GPIOA, MOTOR_2_A_Pin);
GPIO_ResetBits(GPIOA, MOTOR_2_B_Pin);
TIM_SetCompare2(TIM1, pwmRight);
}
else if (pwmRight < 0)
{
GPIO_ResetBits(GPIOA, MOTOR_2_A_Pin);
GPIO_SetBits(GPIOA, MOTOR_2_B_Pin);
TIM_SetCompare2(TIM1, -pwmRight);
}
else
{
GPIO_ResetBits(GPIOA, MOTOR_2_A_Pin);
GPIO_ResetBits(GPIOA, MOTOR_2_B_Pin);
TIM_SetCompare2(TIM1, 0);
}
if (pwmLeft > 0)
{
GPIO_SetBits(GPIOA, MOTOR_3_A_Pin);
GPIO_ResetBits(GPIOA, MOTOR_3_B_Pin);
TIM_SetCompare3(TIM1, pwmLeft);
}
else if (pwmLeft < 0)
{
GPIO_ResetBits(GPIOA, MOTOR_3_A_Pin);
GPIO_SetBits(GPIOA, MOTOR_3_B_Pin);
TIM_SetCompare3(TIM1, -pwmLeft);
}
else
{
GPIO_ResetBits(GPIOA, MOTOR_3_A_Pin);
GPIO_ResetBits(GPIOA, MOTOR_3_B_Pin);
TIM_SetCompare3(TIM1, 0);
}
if (pwmRight > 0)
{
GPIO_SetBits(GPIOA, MOTOR_4_A_Pin);
GPIO_ResetBits(GPIOA, MOTOR_4_B_Pin);
TIM_SetCompare4(TIM1, pwmRight);
}
else if (pwmRight < 0)
{
GPIO_ResetBits(GPIOA, MOTOR_4_A_Pin);
GPIO_SetBits(GPIOA, MOTOR_4_B_Pin);
TIM_SetCompare4(TIM1, -pwmRight);
}
else
{
GPIO_ResetBits(GPIOA, MOTOR_4_A_Pin);
GPIO_ResetBits(GPIOA, MOTOR_4_B_Pin);
TIM_SetCompare4(TIM1, 0);
}
if (pwmLeft > 0)
{
GPIO_SetBits(GPIOA, MOTOR_5_A_Pin);
GPIO_ResetBits(GPIOA, MOTOR_5_B_Pin);
}
else if (pwmLeft < 0)
{
GPIO_ResetBits(GPIOA, MOTOR_5_A_Pin);
GPIO_SetBits(GPIOA, MOTOR_5_B_Pin);
}
else
{
GPIO_ResetBits(GPIOA, MOTOR_5_A_Pin);
GPIO_ResetBits(GPIOA, MOTOR_5_B_Pin);
}
}
// 计算PID控制量
void calculatePID(int *sensorValues)
{
currentError = 0;
int sum = 0;
for (int i = 0; i < 5; i++)
{
if (sensorValues[i] > threshold)
{
currentError += (i - 2) * sensorValues[i];
sum += sensorValues[i];
}
}
if (sum == 0)
{
currentError = 0;
}
else
{
currentError /= sum;
}
integral += currentError;
derivative = currentError - lastError;
lastError = currentError;
pwmLeft = targetSpeed + kp * currentError + ki * integral + kd * derivative;
pwmRight = targetSpeed - kp * currentError - ki * integral - kd * derivative;
}
int main(void)
{
// 初始化GPIO
initGPIO();
// 初始化ADC
RCC_APB2PeriphClockCmd(RCC_APB2Periph_ADC1 | RCC_APB2Periph_ADC2 | RCC_APB2Periph_ADC3 | RCC_APB2Periph_ADC4 | RCC_APB2Periph_ADC5, ENABLE);
ADC_InitTypeDef ADC_InitStruct;
ADC_InitStruct.ADC_Mode = ADC_Mode_Independent;
ADC_InitStruct.ADC_ScanConvMode = ENABLE;
ADC_InitStruct.ADC_ContinuousConvMode = ENABLE;
ADC_InitStruct.ADC_ExternalTrigConv = ADC_ExternalTrigConv_None;
ADC_InitStruct.ADC_DataAlign = ADC_DataAlign_Right;
ADC_InitStruct.ADC_NbrOfChannel = 5;
ADC_Init(ADC1, &ADC_InitStruct);
ADC_Init(ADC2, &ADC_InitStruct);
ADC_Init(ADC3, &ADC_InitStruct);
ADC_Init(ADC4, &ADC_InitStruct);
ADC_Init(ADC5, &ADC_InitStruct);
ADC_RegularChannelConfig(ADC1, ADC_Channel_10, 1, ADC_SampleTime_55Cycles5);
ADC_RegularChannelConfig(ADC2, ADC_Channel_11, 1, ADC_SampleTime_55Cycles5);
ADC_RegularChannelConfig(ADC3, ADC_Channel_12, 1, ADC_SampleTime_55Cycles5);
ADC_RegularChannelConfig(ADC4, ADC_Channel_13, 1, ADC_SampleTime_55Cycles5);
ADC_RegularChannelConfig(ADC5, ADC_Channel_14, 1, ADC_SampleTime_55Cycles5);
ADC_Cmd(ADC1, ENABLE);
ADC_Cmd(ADC2, ENABLE);
ADC_Cmd(ADC3, ENABLE);
ADC_Cmd(ADC4, ENABLE);
ADC_Cmd(ADC5, ENABLE);
ADC_ResetCalibration(ADC1);
ADC_ResetCalibration(ADC2);
ADC_ResetCalibration(ADC3);
ADC_ResetCalibration(ADC4);
ADC_ResetCalibration(ADC5);
while (ADC_GetResetCalibrationStatus(ADC1) || ADC_GetResetCalibrationStatus(ADC2) || ADC_GetResetCalibrationStatus(ADC3) || ADC_GetResetCalibrationStatus(ADC4) || ADC_GetResetCalibrationStatus(ADC5));
ADC_StartCalibration(ADC1);
ADC_StartCalibration(ADC2);
ADC_StartCalibration(ADC3);
ADC_StartCalibration(ADC4);
ADC_StartCalibration(ADC5);
while (ADC_GetCalibrationStatus(ADC1) || ADC_GetCalibrationStatus(ADC2) || ADC_GetCalibrationStatus(ADC3) || ADC_GetCalibrationStatus(ADC4) || ADC_GetCalibrationStatus(ADC5));
ADC_SoftwareStartConvCmd(ADC1, ENABLE);
ADC_SoftwareStartConvCmd(ADC2, ENABLE);
ADC_SoftwareStartConvCmd(ADC3, ENABLE);
ADC_SoftwareStartConvCmd(ADC4, ENABLE);
ADC_SoftwareStartConvCmd(ADC5, ENABLE);
// 初始化定时器
RCC_APB2PeriphClockCmd(RCC_APB2Periph_TIM1, ENABLE);
TIM_TimeBaseInitTypeDef TIM_TimeBaseStruct;
TIM_TimeBaseStruct.TIM_Prescaler = 72 - 1;
TIM_TimeBaseStruct.TIM_CounterMode = TIM_CounterMode_Up;
TIM_TimeBaseStruct.TIM_Period = 1000 - 1;
TIM_TimeBaseStruct.TIM_ClockDivision = TIM_CKD_DIV1;
TIM_TimeBaseStruct.TIM_RepetitionCounter = 0;
TIM_TimeBaseInit(TIM1, &TIM_TimeBaseStruct);
TIM_Cmd(TIM1, ENABLE);
TIM_OCInitTypeDef TIM_OCInitStruct;
TIM_OCInitStruct.TIM_OCMode = TIM_OCMode_PWM1;
TIM_OCInitStruct.TIM_OutputState = TIM_OutputState_Enable;
TIM_OCInitStruct.TIM_OutputNState = TIM_OutputNState_Disable;
TIM_OCInitStruct.TIM_Pulse = 0;
TIM_OCInitStruct.TIM_OCPolarity = TIM_OCPolarity_High;
TIM_OCInitStruct.TIM_OCNPolarity = TIM_OCNPolarity_High;
TIM_OCInitStruct.TIM_OCIdleState = TIM_OCIdleState_Reset;
TIM_OCInitStruct.TIM_OCNIdleState = TIM_OCNIdleState_Reset;
TIM_OC1Init(TIM1, &TIM_OCInitStruct);
TIM_OC2Init(TIM1, &TIM_OCInitStruct);
TIM_OC3Init(TIM1, &TIM_OCInitStruct);
TIM_OC4Init(TIM1, &TIM_OCInitStruct);
while (1)
{
int sensorValues[5];
readSensors(sensorValues);
calculatePID(sensorValues);
controlMotors(pwmLeft, pwmRight);
}
}
```
这个代码中,我们使用了STM32F103C8T6的定时器和PWM功能来控制电机的转速,使用了STM32F103C8T6的ADC功能来读取循迹传感器的值,并通过PID算法计算出左右电机的PWM值,从而实现对五路循迹小车的控制。
stm32f103c8t6用pid算法写一个五路的循迹小车
首先,需要了解PID控制算法的基本原理。PID控制器是一种常见的控制器,它可以通过测量当前状态和目标状态之间的差异来计算输出信号,从而使系统稳定在目标状态。PID控制器由三部分组成:比例项、积分项和微分项,可以通过调整这三个项的权重来优化控制器的性能。
对于循迹小车,需要使用线性光敏二极管(LDR)传感器来检测车辆的位置,然后使用PID控制器来调整车轮的速度,使车辆沿着轨迹行驶。
以下是一个简单的 PID 控制器的实现示例:
```c
#include <stdint.h>
// PID控制器参数
#define KP 0.5
#define KI 0.2
#define KD 0.1
// PID控制器状态
typedef struct {
float error;
float error_sum;
float error_diff;
float last_error;
} pid_state_t;
// PID控制器初始化
void pid_init(pid_state_t *pid) {
pid->error = 0;
pid->error_sum = 0;
pid->error_diff = 0;
pid->last_error = 0;
}
// PID控制器计算输出
float pid_compute(pid_state_t *pid) {
float output = 0;
pid->error_diff = pid->error - pid->last_error;
pid->error_sum += pid->error;
output = KP * pid->error;
output += KI * pid->error_sum;
output += KD * pid->error_diff;
pid->last_error = pid->error;
return output;
}
// 检测传感器状态
uint8_t get_sensor_state(void);
int main(void) {
// 初始化PID控制器
pid_state_t pid;
pid_init(&pid);
// 循迹小车控制循环
while (1) {
// 检测传感器状态
uint8_t sensor_state = get_sensor_state();
// 计算偏差值
float error = /* 根据传感器状态计算偏差值 */;
// 更新PID控制器状态
pid.error = error;
float output = pid_compute(&pid);
// 根据PID输出控制车轮速度
/* 根据输出控制车轮速度 */
}
}
```
在这个示例中,我们使用了一个 `pid_state_t` 结构体来存储 PID 控制器的状态,其中包括当前偏差值、偏差值累加、偏差值差分和上一次偏差值。我们还定义了三个常量 `KP`、`KI` 和 `KD` 来设置比例、积分和微分项的权重。
在循迹小车的控制循环中,我们首先检测传感器状态,然后根据传感器状态计算偏差值。接着,我们更新 PID 控制器的状态,并通过调用 `pid_compute` 函数计算输出值。最后,根据输出值控制车轮速度,使车辆沿着轨迹行驶。
需要注意的是,这只是一个简单的示例,实际应用中需要根据具体的硬件和传感器进行调整。另外,循迹小车的控制循环还需要考虑避障、转向等因素,需要进一步完善和优化。
相关推荐
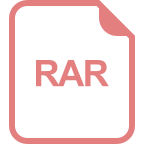
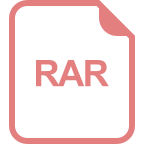
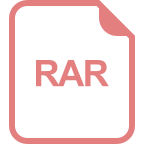
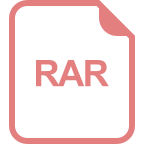
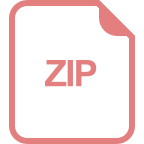
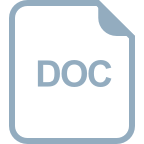
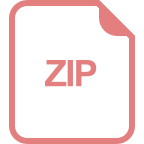
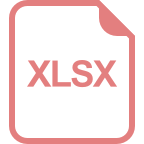
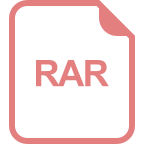
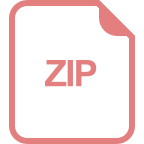
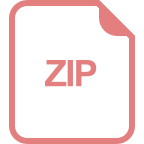
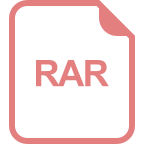
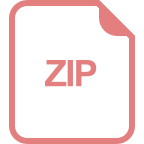
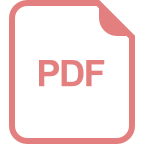