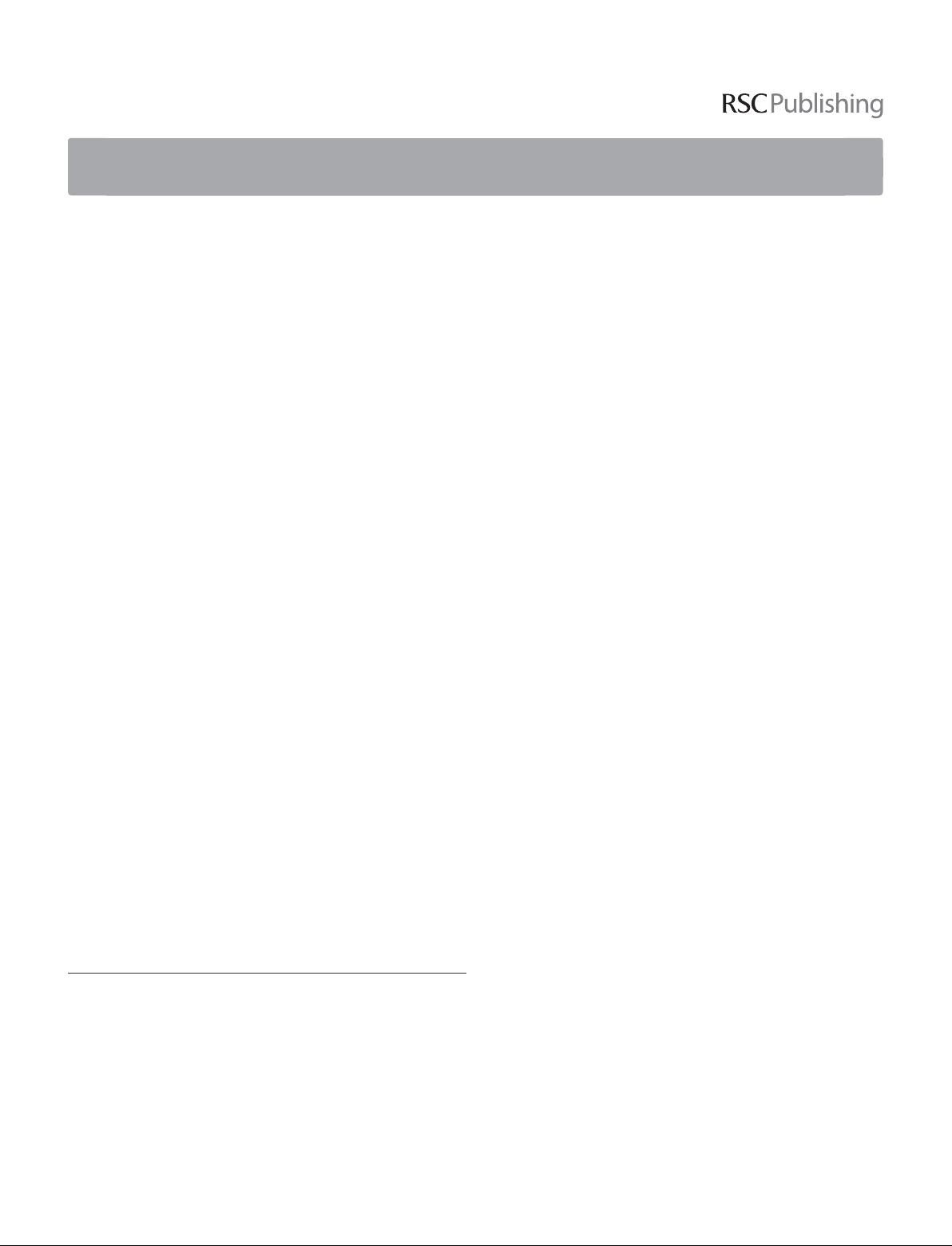
Synthesis of all-deuterated tris(2-phenylpyridine)
iridium for highly stable electrophosphorescence: the
“deuterium effect”†
Ping Wang,‡
a
Fei-Fei Wang,‡
b
Yi Chen,
a
Qiang Niu,
a
Lei Lu,
a
Hong-Ming Wang,
a
Xi-Cun Gao,
*
a
Bin Wei,
*
b
Hong-Wei Wu,
c
Xin Cai
c
and De-Chun Zou
*
c
Device stability and life-time rank the key issues for PhOLEDs. We
synthesized deuterated Ir(ppy)
3
-D
24
. A device based on it has a
current density twenty times higher than and a life-time six times
longer than devices based on Ir(ppy)
3
. The more stable C–Dbondis
found to be the main contributing factor, called the “deuterium
effect”.
Device lifetime and stability at high current densities are the key
issues for phosphorescent organic light-emitting diodes (PhO-
LEDs) because large scale manufacturing of real-world organic
at-panel color displays and white-lighting need thousands of
hours of operating time and are oen used at a high luminance.
Studies have revealed some chemical and physical degradation
mechanisms for PhOLEDs, such as cleavage of substituents of
organic materials,
1
degradation in the HTL (hole-transport
layer),
2
chemical degradation or decomposition of emitter/
host,
3
concentration quenching,
4
charge-trapping problems at
the sites of the dopant molecules owing to the large band gap
difference between the host and dopant molecules,
5
triplet–
triplet annihilation,
6
inefficient host to guest energy transfer,
7
unbalanced carrier injection/transport,
8
exciton diffusion from
the emitting layer,
9
electrochemically instability of the host
10
and electric eld induced quenching.
11
However, the universal
key factors detrimental to PhOLED life-time remain unclear,
especially why the devices suffer “roll-off” under high current
densities.
In PhOLEDs, the phosphorescent materials are usually
doped as emitting guests into charge-transporting and energy
harvesting host materials to obtain higher efficiency and longer
life-time. The presence of a heavy-metal atom in the phospho-
rescent matrix provides a signicant spin-orbit interaction that
allows the decay of a previously spin-forbidden radiative triplet
to happen. Due to both singlet and triplet excited states
participating in the emission, the internal quantum efficiency
can potentially reach as high as 100%.
12
This paves the way for
high-efficiency organic color displays and white-lighting.
Iridium complexes are the most frequently used electro-
phosphorescent materials based on the remarkable “heavy
atom effect” of the Ir
3+
nucleus. From this, early in 2009, we
came up with the idea of “all deuterating” the ligand to obtain a
heavier ligand for iridium for both green and blue electro-
phosphorescence. Close to the end of the synthesis, we
searched to nd a reference and discovered that someone else
had already executed the plan.
13
However, our deuterating
method is di fferent to theirs. Our direct synthesis from simple
reagents creates a higher deuteration rate (more than 99%
compared to the 95% reported). More importantly, we have
fabricated and tested electrophosphorescent devices. In the
following paragraphs, we will show that our device test results
have contributed to understanding the most important issues:
the device stability and operating lifetime can be greatly
increased or extended and the factors for these improvements
are identied.
By starting from the very simple and inexpensive reagent,
deuterated benzene, we obtained deuterated bromobenzene,
and then by reacting deuterated bromobenzene with another
simple and inexpensive regent, deuterated pyridine, we
obtained deuterated 2-phenylpyridine. Aer that, we obtained
deuterated tris(2-phenylpyridine)iridium by using this ligand.
We initially hoped that through deuteration of 2-phenyl-
pyridine, we could greatly increase the phosphorescence effi-
ciency of the iridium complex as a whole by introducing twenty
four heavier deuterium atoms instead of the hydrogen atom and
then greatly improve the device efficiency. However, contrary to
a
Department of Chemistry, School of Science, Nanchang University, Xue-Fu Road 999,
Hong-Gu-Tan New District, Nanchang 330031, China. E-mail: xcgao@ncu.edu.cn
b
Key Laboratory of Advanced Display and System Applications, Ministry of Education,
Shanghai University, Shanghai 200072, China. E-mail: bwei@shu.edu.cn
c
Beijing National Laboratory for Molecular Sciences, Key Laboratory of Polymer
Chemistry and Physics of Ministry of Education, College of Chemistry and Molecular
Engineering, Peking University, Beijing 100871, China. E-mail: dczou@pku.edu.cn
† Electronic supplementary information (ESI) available. CCDC 917827. For ESI
and crystallographic data in CIF or other electronic format see DOI:
10.1039/c3tc30547c
‡ These authors contributed equally to this work.
Cite this: J. Mater. Chem. C, 2013, 1,
4821
Received 24th March 2013
Accepted 2nd July 2013
DOI: 10.1039/c3tc30547c
www.rsc.org/MaterialsC
This journal is ª The Royal Society of Chemistry 2013 J. Mater. Chem. C, 2013, 1, 4821–4825 | 4821
Journal of
Materials Chemistry C
COMMUNICATION
Published on 02 July 2013. Downloaded on 10/12/2013 08:56:20.