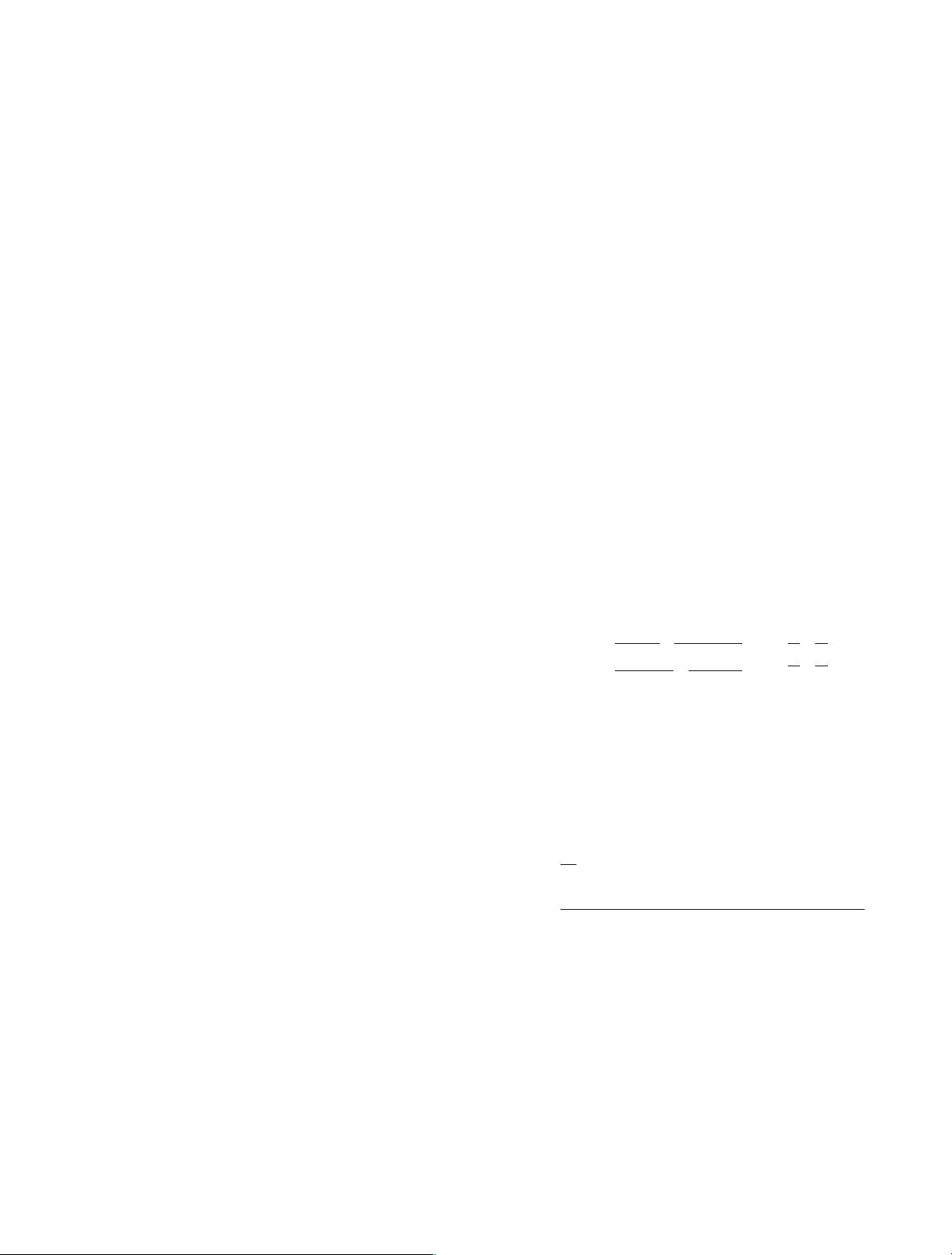
Optical pulse repetition rate multiplication based
on series-coupled double-ring resonator
Xiaowei Dong,* Mengzhen Xu, and Qiangqiang Zhang
College of Information Engineering, North China University of Technology, Beijing 100144, China
*Corresponding author: way7803@163.com
Received September 30, 2015; revised December 24, 2015; accepted January 10, 2016;
posted February 12, 2016 (Doc. ID 251088); published March 21, 2016
In this paper, optical pulse repetition rate multiplication based on a series-coupled double-ring resonator is pro-
posed. First, the spectral characteristic of the series-coupled double-ring resonator is simulated and the optimum
coupling coefficients to achieve a periodic flat-top passband are obtained. Then, high-quality pulse repetition rate
multiplication is realized by periodically filtering out spectral lines of the input pulse train. Different multipli-
cation factors N 2, 3, 4, 5 can be obtained by adjusting the ring radii. In addition, compared with a single-ring
resonator, the multiplied output pulse train by a series-coupled double-ring resonator exhibits much better power
uniformity. © 2016 Chinese Laser Press
OCIS codes: (130.3120) Integrated optics devices; (230.4555) Coupled resonators; (230.7408) Wavelength
filtering devices.
http://dx.doi.org/10.1364/PRJ.4.000061
1. INTRODUCTION
With the recent development of ultrahigh-speed optical com-
munication systems, techniques for generating and processing
high repetition rate optical pulse trains are attracting consid-
erable interest [1]. Since conventional mode-locking or direct-
modulating methods are hard to operate at such ultrahigh
speed, optical pulse repetition rate multiplication (PRRM) be-
comes necessary [2]. Most PRRM schemes are based on peri-
odically changing the amplitude and/or phase of the input
pulse spectrum. For example, by spectral amplitude filtering,
a double-passing Fabry–Perot filter [3], an array waveguide
grating [4], and a lattice-form Mach–Zehnder interferometer
[5] have been used for generating high repetition rate optical
pulse trains. For phase-filtering-based PRRM, chirped-fiber-
Bragg gratings [6] have been employed to implement the
fractional temporal Talbot effect. Due to the merits of small
size and compatibility with the integration process, ring-
resonator-based optical PRRM exhibits better applicable pros-
pects. In Ref. [7], optical pulse trains are generated by using a
multiple-microring-based time interleaved architecture. In
Refs. [8,9], doubling and quadrupling PRRM are obtained
by microring-induced beating resonance. In Ref. [10], PRRM
and shaping are achieved by ring resonator arrays. However,
device fabrication and stability become more difficult as the
number of ring resonators increases. Therefore, in this paper,
by simulating the spectral characteristic of the series-coupled
double-ring resonator, we propose a much more compact
optical PRRM with better power uniformity.
2. THEORETICAL BACKGROUND
Figure 1(a) is a typical configuration of a series-coupled
double-ring resonator. The transfer function can be obtained
by dividing the series-coupled double-ring resonator into three
directional couplers (coupling coefficients are K
0
, K
1
, and
K
2
) and two uncoupled half-rings (ring radii are R
1
and R
2
,
respectively), as shown in Figs. 1(b) and 1(c).
Based on the transfer matrix method, the transfer function
of a series-coupled double-ring resonator can be obtained
as [11]
G −j
8
<
:
Y
k1;2
2
4
τ
1∕2
k
·e
jβ·πR
k
K
k
−
τ
−1∕2
k
·e
−jβ·πR
k
·T
k
K
k
τ
1∕2
k
·e
jβ·πR
k
·T
k
K
k
−
τ
−1∕2
k
·e
−jβ·πR
k
K
k
3
5
9
=
;
·
"
1
K
0
−
T
0
K
0
T
0
K
0
−
1
K
0
#
; (1)
where β 2π · n
eff
∕λ is the propagating constant, n
eff
is
the effective refractive index, λ is the wavelength, τ
k
exp−α · 2πR
k
is the waveguide normalized loss, and T
k
and
K
k
are the normalized transferring coefficient and coupling
coefficient, which have the relationship T
2
k
K
2
k
1.
By assuming no input signal at port 3 (E
3
0) and defining
one-round phase within the ring as ϕ
1;2
2π · n
eff
∕λ · 2πR
1;2
,
the spectral responses at drop port Dλ can be obtained as
Dλ
E
4
E
1
jK
1
K
2
K
3
τ
1∕2
1
τ
1∕2
2
e
jϕ
1
ϕ
2
∕2
1 − T
1
T
2
τ
1
e
jϕ
1
T
2
T
3
τ
2
e
jϕ
2
T
1
T
3
τ
1
τ
2
e
jϕ
1
ϕ
2
.
(2)
Due to the resonant characteristic, Dλ is periodic with re-
spect to the inverse of wavelength. Defining FSR λ
m1
− λ
m
as the periodic resonant spacing and using the inverse Fourier
transform (F
−1
), the temporal domain impulse response of
Dt is discrete-time, as shown by [5]
Dt
X
∞
p−∞
F
−1
Dλj
tp∕FSR
· δt − p∕FSR. (3)
When a periodic input pulse train a
in
t
P
∞
q−∞
a
0
t − qT
(where a
0
t represents the complex envelope of an individual
pulse and T is the temporal period) is injected into port 1, the
Dong et al. Vol. 4, No. 2 / April 2016 / Photon. Res. 61
2327-9125/16/020061-04 © 2016 Chinese Laser Press