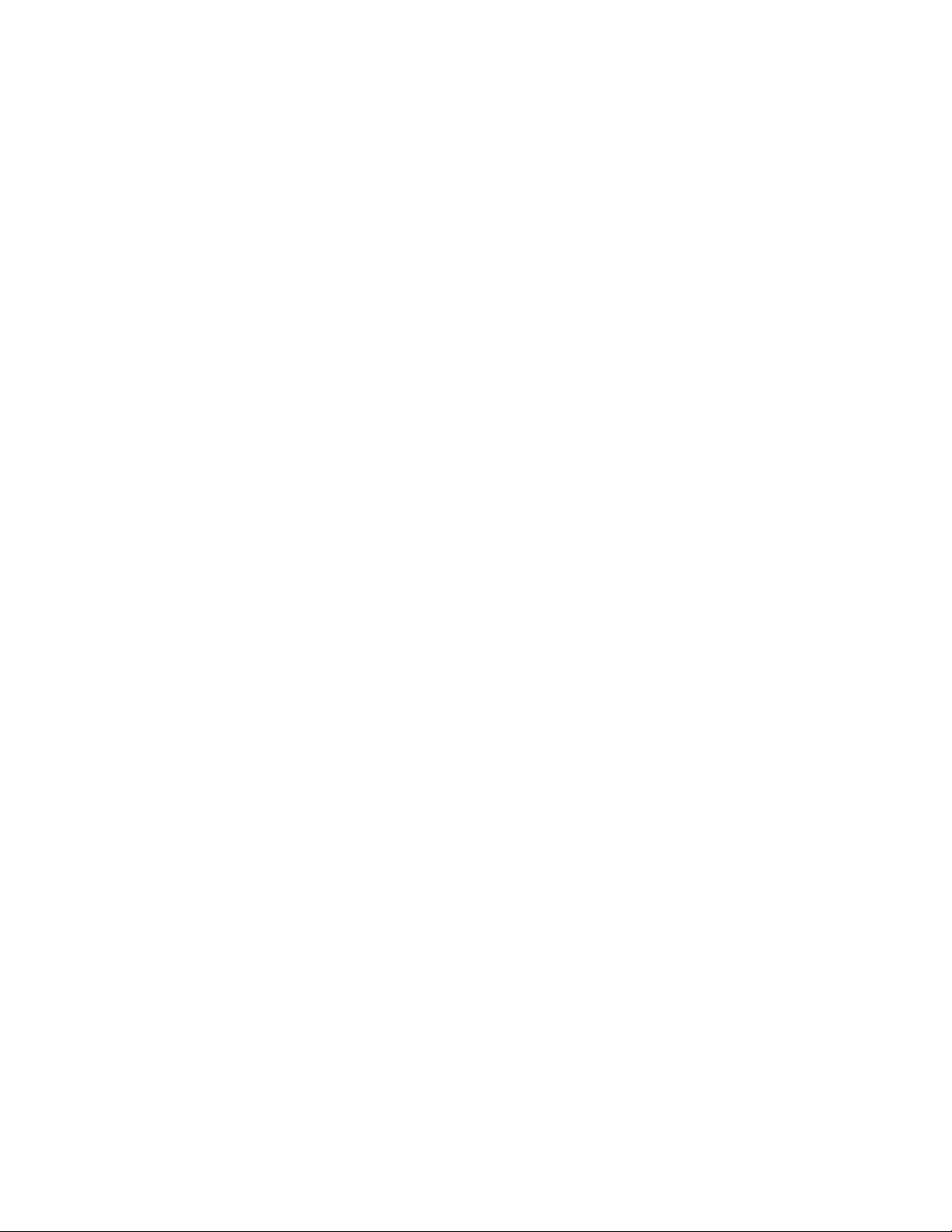
Non-Orthogonal Multiple Access (NOMA)
for Cellular Future Radio Access
Yuya Saito
*)
, Yoshihisa Kishiyama
*)
, Anass Benjebbour
*)
, Takehiro Nakamura
*)
, Anxin Li
**)
, and Kenichi Higuchi
***)
*)
Radio Access Network Development Department, NTT DOCOMO, INC.
**)
DOCOMO Beijing Communications Laboratories Co., Ltd.
***)
Graduate School of Science and Technology, Tokyo University of Science
E-mail: yuuya.saitou.fa@nttdocomo.com
Abstract—This paper presents a non-orthogonal multiple access
(NOMA) concept for cellular future radio access (FRA) towards the
2020s information society. Different from the current LTE radio
access scheme (until Release 11), NOMA superposes multiple users
in the power domain although its basic signal waveform could be
based on the orthogonal frequency division multiple access
(OFDMA)
or the discrete Fourier transform (DFT)-spread OFDM the
same as LTE baseline. In our concept, NOMA adopts a successive
interference cancellation (SIC) receiver as the baseline receiver
scheme for robust multiple access, considering the expected
evolution of device processing capabilities in the future. Based on
system-level evaluations, we show that the downlink NOMA with
SIC improves both the capacity and cell-edge user throughput
performance irrespective of the availability of the frequency-selective
channel quality indicator (CQI) on the base station side. Furthermore,
we discuss possible extensions of NOMA by jointly applying multi-
antenna/site technologies with a proposed NOMA/MIMO scheme
using SIC and an interference rejection combining (IRC) receiver to
achieve further capacity gains, e.g., a three-fold gain in the spectrum
efficiency representing a challenging target for FRA.
Keywords
−
NOMA, non-orthogonal, interference cancellation,
multiple access, future radio access
I. INTRODUCTION
Radio access technologies for cellular mobile communications are
typically characterized by multiple access schemes, e.g., frequency
division multiple access (FDMA), time division multiple access
(TDMA), code division multiple access (CDMA), and OFDMA. In
the 3.9 and 4th generation (4G) mobile communication systems such
as Long-Term Evolution (LTE) [1] and LTE-Advanced [2, 3],
standardized by the 3rd Generation Partnership Project (3GPP),
orthogonal multiple access based on OFDMA or single carrier (SC)-
FDMA is adopted. Orthogonal multiple access was a reasonable
choice for achieving good system-level throughput performance in
packet-domain services with simple single-user detection.
However, considering future radio access (FRA) in the 2020s,
further enhancement to achieve significant gains in capacity and
system throughput performance is a high priority requirement in view
of the recent exponential increase in the volume of mobile traffic, e.g.,
beyond a 500 fold increase in the next decade [4], and the need for
enhanced delay-sensitive high-volume services such as video
streaming and cloud computing. Thus, the 3GPP recently has
initiated discussions on further evolution of LTE towards the future,
i.e., Release 12 and onwards. In order to continue to ensure the
sustainability of 3GPP radio access technologies over the coming
decade, new solutions must be identified and provided that can
respond to future challenges [4, 5]. Also, recent trends in research
activity for the next generation of mobile and wireless
communication systems for 2020 and beyond have emerged such as
the Mobile and wireless communications Enablers for the 2020
Information Society (METIS) project [6].
To accommodate such demands, a combination of multiple
approaches, i.e., technologies for spectrum efficiency enhancement,
spectrum extension with efficient use of higher frequency bands, and
network densification deploying small cells would be required. In
this sense, innovative radio access technologies to enhance
significantly the spectrum efficiency and the small-cell enhancement
now on-going in the 3GPP are very important [7]. Although it may
be very challenging, our target for FRA toward the 2020s is to
achieve a further 3-fold enhancement in the spectrum efficiency
compared to the LTE baseline. Since LTE has already achieved a 3-4
fold enhancement in the spectrum efficiency compared to 3G High-
Speed Packet Access (HSPA), our target gain would be more than 10
fold compared to the 3G HSPA. Thus, for instance, the gain in the
total capacity such as beyond 500 fold can be achieved if other ways,
i.e., spectrum extension using higher frequency bands and network
densification achieve a capacity gain of 50 fold.
To enhance the spectrum efficiency, we have proposed a non-
orthogonal multiple access (NOMA) scheme using interference
cancellation, which is a promising candidate as a new cellular
multiple access scheme for FRA [8-12]. Here, FRA may include
future LTE enhancements, i.e., beyond Release 12, and potential new
systems beyond 4G. In the paper, we present our NOMA concept for
FRA with some technical considerations and proposals with results
from system-level evaluations. The remainder of the paper is
organized as follows. Section II presents our motivations in
proposing the NOMA concept. Section III describes the principle
behind the basic NOMA scheme using a successive interference
cancellation (SIC) receiver and its power allocation scheme with
some system-level evaluations. In Section IV, we discuss possible
extensions of NOMA by applying the multi-antenna/site technologies
with a proposed NOMA/Multiple-Input Multiple-Output (MIMO)
scheme [10] to achieve a further capacity gain. Finally, Section V
concludes the paper.
II. MOTIVATIONS FOR NOMA CONCEPT
Table I shows the multiple access schemes for 3G (W-
CDMA/HSPA), 3.9/4G (LTE/LTE-Advanced), and our expected
FRA for the 2020s. In 3G, non-orthogonal user multiplexing based
on direct sequence (DS)-CDMA is used. The receiver uses simple
single-user detection such as the Rake receiver. Fast transmission
power control (TPC) is adopted to address the well-known near-far
problem in cellular deployments due to its non-orthogonal property.
In 3.9/4G, orthogonal user multiplexing based on OFDMA or SC-
FDMA is adopted to achieve higher throughput performance in
packet-domain services. Its signal waveform such as orthogonal
frequency division multiplexing (OFDM), including discrete Fourier
transformation (DFT)-spread OFDM [1], provides important benefits,
i.e., robustness against multipath interference and good affinity to
MIMO technologies, that dramatically increase the achievable data
rate. Furthermore, fast TPC becomes non-essential because there is
no near-far problem in orthogonal user multiplexing. Adaptive
978-1-4673-6337-2/13/$31.00 ©2013 IEEE