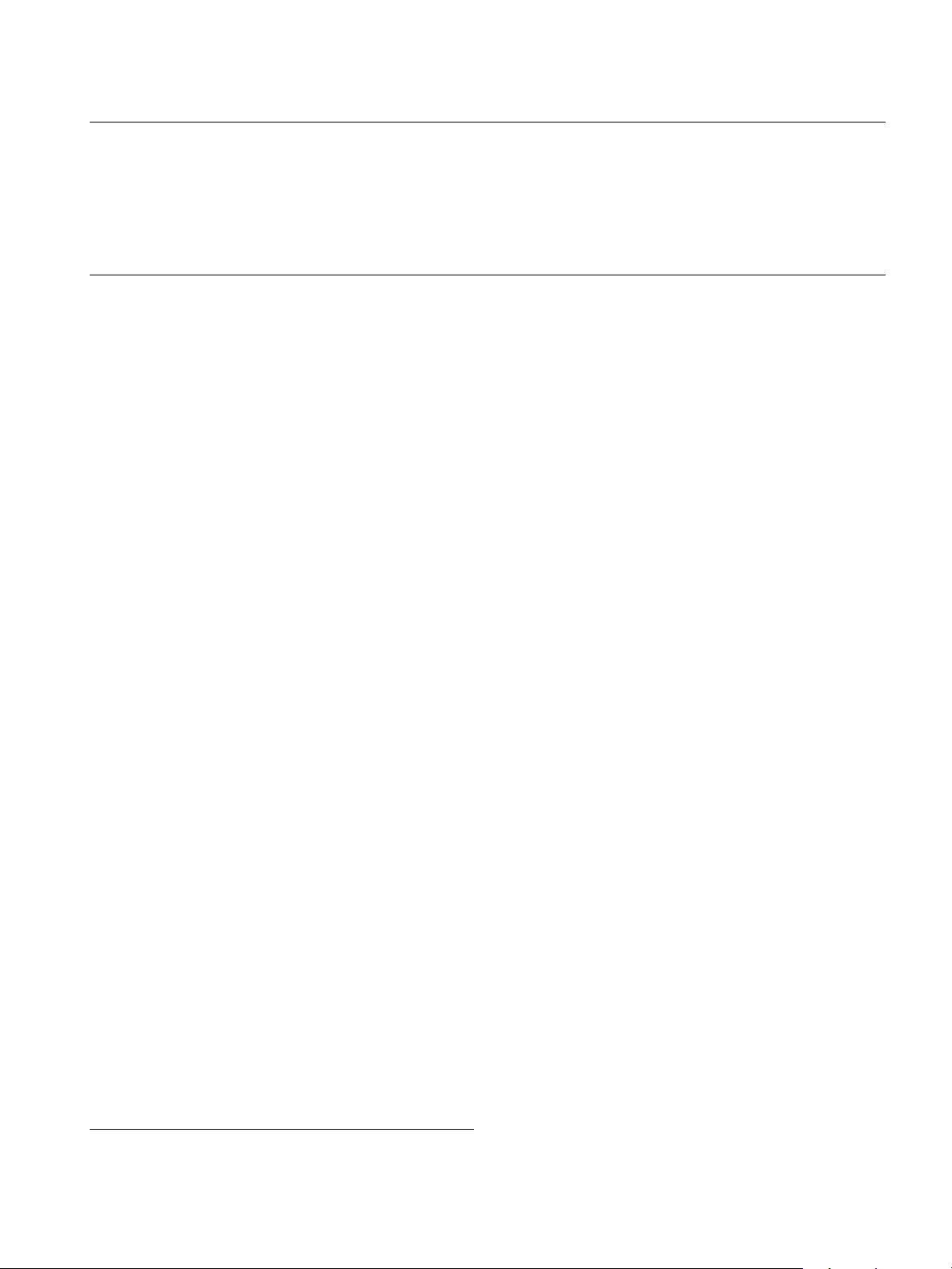
High Power Laser Science and Engineering, (2018), Vol. 6, e11, 9 pages.
© The Author(s) 2018. This is an Open Access article, distributed under the terms of the Creative Commons Attribution licence (http://creativecommons.org/
licenses/by/4.0/), which permits unrestricted re-use, distribution, and reproduction in any medium, provided the original work is properly cited.
doi:10.1017/hpl.2018.3
Scaling diode-pumped, high energy picosecond lasers to
kilowatt average powers
Brendan A. Reagan
1,2
, Cory Baumgarten
3
, Elzbieta Jankowska
1
, Han Chi
1
, Herman Bravo
1,2
, Kristian
Dehne
2
, Michael Pedicone
3
, Liang Yin
1
, Hanchen Wang
3
, Carmen S. Menoni
1,2
, and Jorge J. Rocca
1,2,3
1
Department of Electrical and Computer Engineering, Colorado State University, Fort Collins, CO 80523, USA
2
XUV Lasers Inc., PO Box 273251, Fort Collins, CO 80527, USA
3
Department of Physics, Colorado State University, Fort Collins, CO 80523, USA
(Received 3 December 2017; accepted 3 January 2018)
Abstract
Recent results in the development of diode-driven high energy, high repetition rate, picosecond lasers, including the
demonstration of a cryogenic Yb:YAG active mirror amplifier that produces 1.5 J pulses at 500 Hz repetition rate
(0.75 kW average power) are reviewed. These pulses are compressed resulting in the generation of ∼5 ps duration,
1 J pulses with 0.5 kW average power. A full characterization of this high power cryogenic amplifier, including at-
wavelength interferometry of the active region under >1 kW average power pump conditions, is presented. An initial
demonstration of operation at 1 kW average power (1 J, 1 kHz) is reported.
Keywords: advanced laser technology and applications; diode-pumped solid-state laser and applications; high power laser; high power laser
related laser components; laser amplifiers
1. Introduction
The continued advancement of compact, short pulse lasers
with high average power is crucial for the development of
important applications. These include driving the next gen-
eration of ultra-intense few-cycle and single-cycle sources
at wavelengths ranging from the visible to the far infrared
through optical parametric chirped pulse amplification
(OPCPA)
[1]
and related schemes such as frequency-domain
optical parametric amplification (FOPA)
[2]
. These lasers
are also in demand for pumping tabletop secondary sources
of radiation at more extreme wavelengths, such as drivers
for coherent and incoherent sources of extreme ultraviolet
(EUV), soft X-ray and hard X-rays
[3–8]
, and, at the opposite
end of the spectrum, terahertz sources exploiting techniques
like optical rectification
[9, 10]
. These compact coherent, short
wavelength sources can be realized through high harmonic
generation
[5, 6]
and plasma-based soft X-ray lasers
[7, 8]
. In
turn, the development of high power incoherent, plasma-
based sources of EUV radiation is key to the advancement
of lithography for the fabrication of the next generations
Correspondence to: B. A. Reagan, Colorado State University, USA.
Email: brendan.reagan@colostate.edu
of computer processors and memory
[3]
. Furthermore,
high flux beams of high energy picosecond pulses are of
interest for the production of gamma rays through inverse
Compton scattering with accelerated electron beams
[11, 12]
.
In addition, these lasers are of interest for the generation
of high energy femtosecond laser pulses at very high
repetition rates (multi-kilowatt-average powers) to enable
the implementation of compact, practical particle acceler-
ators based on laser wakefield acceleration
[13, 14]
, greatly
increasing access to giga electron-volt beams for basic
research and therapeutic radiation treatments. These and
other applications are motivating the development of high
average power, short pulse lasers by groups at institutions
worldwide.
Figure 1 summarizes the current state of the art of high
energy, high average power, diode-pumped short pulse
lasers
[15–38]
. Considerable progress has been made in the
development of picosecond and femtosecond lasers with
pulse energies of tens of millijoules to a few hundred mil-
lijoules at average powers approaching or exceeding 1 kW.
These lasers have been enabled using various techniques.
The thin disk geometry
[39]
was particularly successful, and
was recently employed to generate >200 mJ pulses at 5 kHz
repetition rate and >100 mJ at 10 kHz repetition rate
[25]
.
Other schemes include the innoslab geometry
[40]
, which is
1