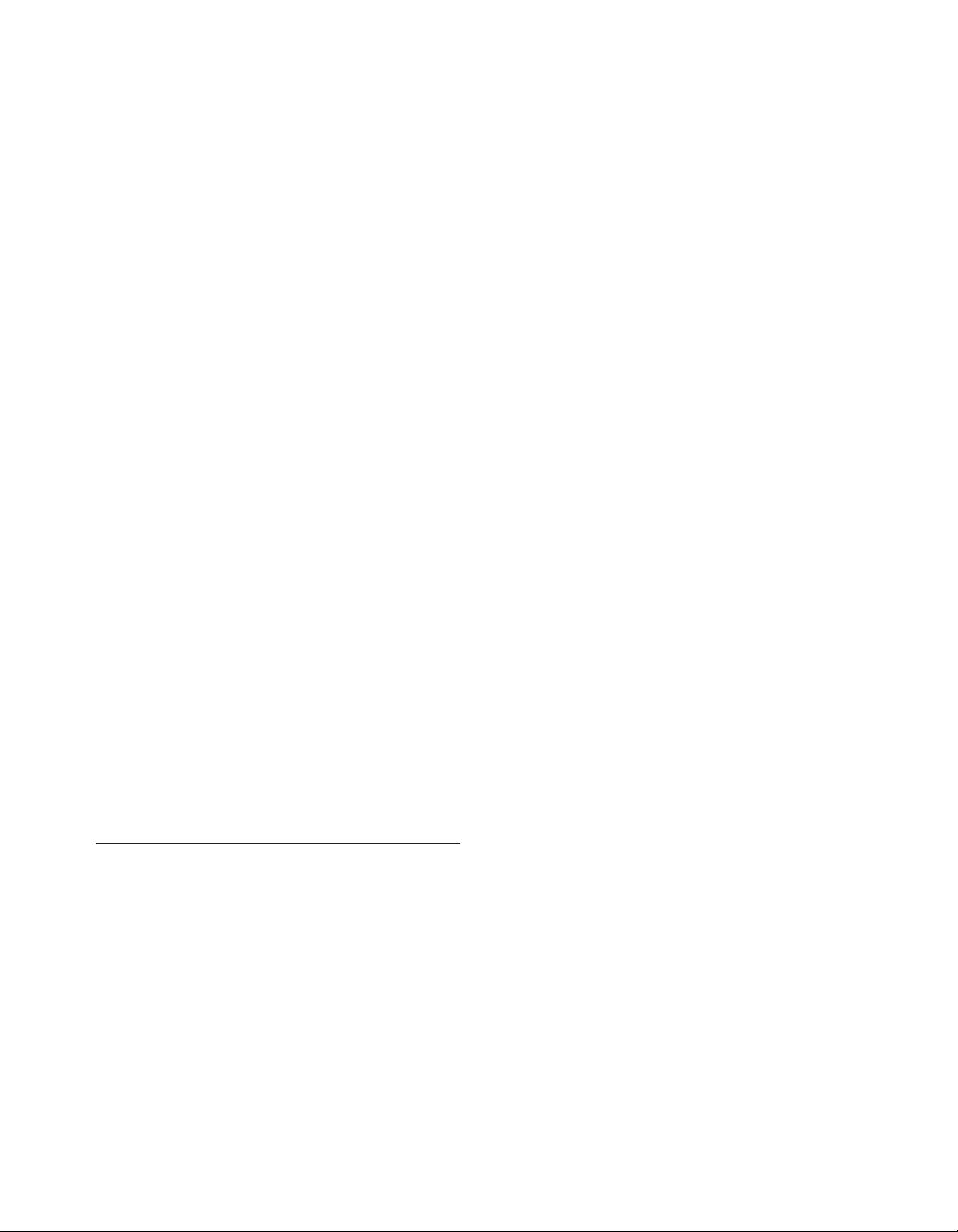
2. G.V. Eleftheriades and G.M. Rebeiz, Self and mutual admittance of
slot antennas on a dielectric half-space, Int J Infrared Millimeter
Waves 14 (1993), 1925–1946.
3. F. Elek, R. Abhari, and G.V. Eleftheriades, A uni-directional ring-
slot antenna achieved by using an electromagnetic band-gap surface,
IEEE Trans Antennas Propag 53 (2005), 181–190.
4. C. Lo
€
ucker, T. Vaupel, and T.F. Eibert, Radiation efficient unidirec-
tional low-profile slot antenna elements for x-band application, IEEE
Trans Antennas Propag 53 (2005), 2765–2768.
5. Y. Yoshimura, A microstripline slot antenna (short papers), IEEE
Trans Microwave Theory Tech 20 (1972), 760–762.
6. M. Qiu, M. Simcoe, and G.V. Eleftheriades, Radiation efficiency of
printed slot antennas backed by a ground reflector, In: 2000 IEEE
Antennas and Propagation Society International Symposium, IEEE,
2000, pp. 1612–1615.
7. J. Jacobs, Self and mutual admittance of cpw-fed slots on conductor-
backed two-layer substrate, Microwave Opt Technol Lett 49 (2007),
2798–2802.
8. F.-R. Yang, K.-P. Ma, Y. Qian, and T. Itoh, A novel tem waveguide
using uniplanar compact photonic-bandgap (uc-pbg) structure, IEEE
Trans Microwave Theory Tech 47 (1999), 2092–2098.
9. D. Sievenpiper, L. Zhang, R.F. Broas, N.G. Alexopolous, and E.
Yablonovitch, High-impedance electromagnetic surfaces with a for-
bidden frequency band, IEEE Trans Microwave Theory Tech 47
(1999), 2059–2074.
10. R. Abhari and G.V. Eleftheriades, Metallo-dielectric electromagnetic
bandgap structures for suppression and isolation of the parallel-plate
noise in high-speed circuits, IEEE Trans Microwave Theory Tech 51
(2003), 1629–1639.
11. L. Brioullin, Wave propagation in periodic structures, Dover, New
York, 1953.
12. J. Sohn, K.Y. Kim, H.-S. Tae, and H. Lee, Comparative study on
various artficial magnetic conductors for low-profile antenna, Prog
Electromagn Res 61 (2006), 27–37.
13. J.D. Shumpert, W.J. Chappell, and L.P. Katehi, Parallel-plate mode
reduction in conductor-backed slots using electromagnetic bandgap
substrates, IEEE Trans Microwave Theory Tech 47 (1999), 2099–
2104.
14. J.Y. Park, C.C. Chang, Y. Qian, and T. Itoh, An improved low-
profile cavity-backed slot antenna loaded with 2d uc-pbg reflector,
vol.194, In: 2001 IEEE Antennas and Propagation Society Interna-
tional Symposium, IEEE, 2001, pp. 194–197.
15. J. Joubert, J. Vardaxoglou, W.G. Whittow, and J.W. Odendaal, Cpw-
fed cavity-backed slot radiator loaded with an amc reflector, IEEE
Trans Antennas Propag 60 (2012), 735–742.
16. M.E. de Cos and F. Las-Heras, Dual-band uniplanar cpw-fed monop-
ole/ebg combination with bandwidth enhancement, IEEE Antennas
Wireless Propag Lett 11 (2012), 365–368.
V
C
2015 Wiley Periodicals, Inc.
NOVEL MULTIPULSES TECHNOLOGY
FOR PULSE LASER RADAR
Xiangcheng Chen,
1
Keyi Wang,
1
Tao Mei,
2
and
Shaoping Wang
2
1
University of Science and Technology of China, Hefei, China
2
Hefei Institutes of Physical Science, Chinese Academy of Sciences,
Hefei, China; Corresponding author: tmei@iim.ac.cn
Received 15 December 2014
ABSTRACT: A novel unequal spacing multipulses technology for pulse
Laser Radar was proposed in this article. Data accumulation technology
is an efficient method to improve signal-to-noise ratio due to different
statistical characteristics between the echo signal and the noise. Tradi-
tional multipulses technology using equal spaces multipulses always
leads to outputs once the time window has pulses no matter the number
of pulses. We proposed a method using unequal spaces multipulses tech-
nology to improve the poor performance of the equal space multipulses
technology, that is, if and only if the time window had special number
pulses, the novel system would have output signal. We discussed the
method to generate unequal spacing multipulses serials first, and then
the algorithm to deal with the echo signals was also illustrated. Both
simulation and experimental results showed the validity of our algo-
rithm.
V
C
2015 Wiley Periodicals, Inc. Microwave Opt Technol Lett
57:1614–1620, 2015; View this article online at wileyonlinelibrary.com.
DOI 10.1002/mop.29178
Key words: laser radar; signal-to-noise ratio; data accumulation; mul-
tipulses technology
1. INTRODUCTION
Laser radar (Ladar) is a valuable tool for three dimensional
imaging of intensity, velocity, range, thus, it has been widely
used in many applications, such as civilian and military applica-
tions [1–3]. Two key points in the applications of Ladar are the
range measurement capability and eyesafe.
To increase the maximum sensing ranges, we have to
research the strategies about how to improve signal-to-noise
ratio (SNR) for the Ladar system. Three approaches can be
expected to be exploited: (1) increasing the laser output power,
(2) improving the detector or its associated optics, and (3)
improving the modulation scheme [4]. During the last years,
much effort has been made to develop eyesafe rangefinders
based on using singe pulse of very high intensity, but at a wave-
length which is not dangerous for the human eye. With lasers of
this kind range, measurement capabilities of 10 Km or more
have been obtained. Also, some progresses have been made in
improving the detectivity of semiconductor detectors about 1
lm, but with a great cost. Thus, a better approach is urgently
needed to improve modulation schemes.
For continuous laser, there have been many studies focusing
on random modulation laser radar [4–11]. However, those tech-
nologies all need extra optical modulators which are very expen-
sive for now. Another way is utilizing multipulses accumulation
technologies [12,13] to enhance SNR. No need of expensive
modulator makes this technologies advantage in some domains.
Traditional multipulse technologies using equal space pulse
accumulation can enhance SNR, but may lead to false alarm,
thus needing complex algorithm to identify main peak of data
accumulation. In this article, we concentrate on a new unequal
spacing multipulsed technology which can not only preserve the
advantages of traditional multipulse technologies but also can
eliminate the false alarm due to equal space pulse accumulation.
2. THEORETICAL BACKGROUND
2.1. Ladar Detection Model
The signal received by the Ladar receiver contains noises, which
in the bandwidth of the signal will restrict the detection ability
of the Ladar. Therefore, the detection of the signal becomes a
statistical problem. Generally, the Neyman–Pearson criteria [14]
are used to detect the Ladar echo signal in noises. Therefore, we
need to figure out the noise model first.
There have been many noise distributions in many previous
papers [15]. Gaussian distribution with zero-mean are often sup-
posed as the distribution of the noise in the Ladar receiver after
the intermediate frequency amplifier when Ladar uses a linear
Avalanche Photodiodes (APD) as photoelectric detector [16]. In
this article, we also adopt the Gaussian distribution as the noise
distribution, that is, X Nð0; r
2
Þ, then
1614 MICROWAVE AND OPTICAL TECHNOLOGY LETTERS / Vol. 57, No. 7, July 2015 DOI 10.1002/mop