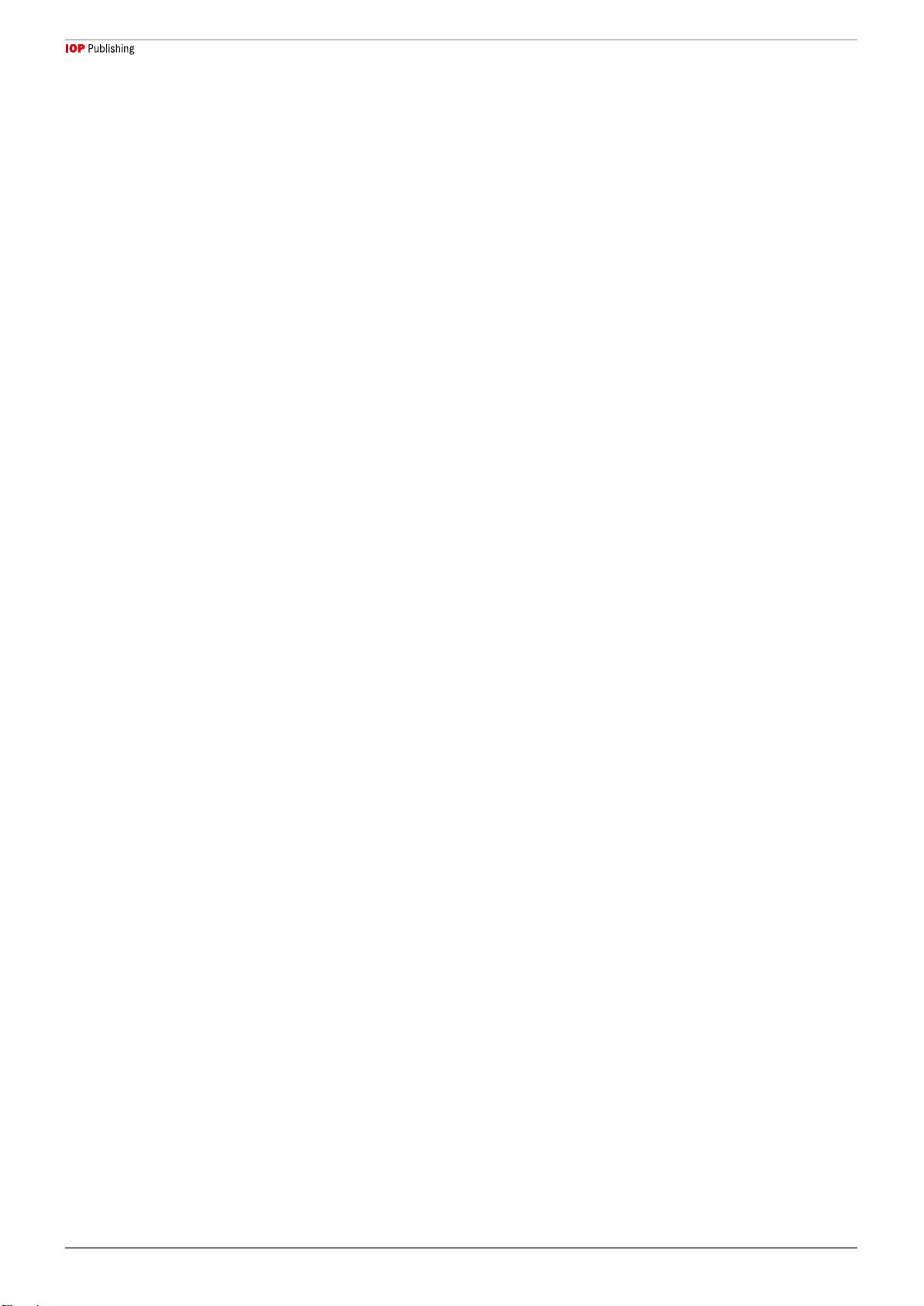
commands (see section 2.2.1). Coordination of loco-
motor organs is generated by interaction between
these controllers, i.e., for the two arms and two legs for
humanoids, the four legs for mammals and the six legs
for insects. For most forms of locomotion the
locomotor organs are segmented, e.g. the trunk in fish
or the legs of animals. This is reflected by the fact that
the relevant controllers are subdivided in neural
networks for each segment (see below). The output of
the controllers, finally, drives the muscles and thereby
generates the locomotor movements. It is important
to note that the impression of the neural structures
underlying the generation of locomotion to be exclu-
sively hierarchical is misleading: all levels of processing
receive marked feedback from the subsequent levels
(figure 1). The contribution of downstream neural
control becomes particularly apparent in view of new
findings on the neural mechanisms for gaze-stabiliza-
tion in vertebrate locomotion: in the anuran tadpole
during locomotion the extra-ocular nuclei are under
the control of ascending rhythmic neural activity,
which originates from the locomotor CPGs (see
section 2.2.1) in the spinal cord (Lambert et al 2012).
Today ample information is available on the loca-
tion of the neural networks in the CNS of animals that
contribute to the functions mentioned above. In verte-
brates, for example, the sequence of levels involved
from decision making to the generation of movements
of the legs, spans from cortical inputs to the basal
ganglia, their disinhibition of particular neurons in the
di- and mesencephalic locomotor regions, the activa-
tion of the reticulospinal system to the activation of
the CPGs in the spinal cord at the level of the loco-
motor organs. Detailed information on the state of
knowledge can be found in various review articles and
text books (Grillner 2006, Orlovsky et al 1999, Kalaska
and Drew 1993). Even though the gross anatomical
organization of the neural networks involved is well-
understood, knowledge is fairly limited on the detailed
topology and operation of the contributing neural net-
works in the control of legged locomotion, for exam-
ple, with respect to the explicit mechanisms in charge
of mediating the above named functions. Among oth-
ers, three issues are open as of today: how does the ner-
vous system selectively control movement and posture
in locomotion simultaneously? Which neural compo-
nents in the local controller networks are targeted by
the command systems? How do primates differ from
other mammals on the mechanistic level by the cor-
tical contribution to locomotor function?
2.2. Organization of the controller architecture for
stepping
In the following sections the role of sensory feedback
will be reviewed for all four phases forming a stepping
cycle for well-studied two, four and six legged animals.
It will become clear that general principles underlie
the generation of the functional stepping motor
output of an animal leg and that sensory feedback
constitutes a major contribution.
2.2.1. Intrinsic factors: CPGs
It has been known for more than a century that there
are neural networks in the CNS of walking animals, so
called central pattern generators (CPGs, see figure 3 ),
which can generate a basic rhythm in the motoneurons
innervating leg muscles. It is characterized by alternat-
ing activity between the antagonistic sets of neurons
associated with the stance and the swing phase (Brown
(1911); for reviews see Delcomyn et al (1981), Grillner
(1981), Pearson et al (2000), Büschges et al (
2011);
most recent comprehensive summary in Orlovsky et al
(1999)). Today information on the topological organi-
zation of the neural networks underlying walking
pattern generation is still not complete, but funda-
mental organizational features of those networks are
known. The most comprehensive knowledge for
locomotor networks in general are available for
lamprey swimming (figure 4(a)) and locust flight
(summary in Orlovsky et al 1999, Büschges et al 2011):
many well studied CPGs for locomotion share (1) a
topology that ensures via mutual inhibition the alter-
nating activity between networks of neurons in charge
of driving the pools of motoneurons, the so-called
‘half-center’ organization (figures 3, 4(a)). They also
share (2) their location close to the innervated
appendage, i.e., networks controlling stepping in
vertebrate hind legs reside in the lumbar spinal cord
whereas networks controlling front leg movement
reside in the cervical spinal cord (for review see
Grillner (1981)). Networks controlling an insect mid-
dle leg reside in the mesothoracic ganglion (for review
see Ritzmann and Büschges (2007a)). In vivo the CPGs
can be brought to generate rhythmic motor activity by
descending pathways from brain centers, like the
mesencephalic locomotor region in vertebrate walking
(for review see Orlovsky et al (1999)). In vitro the CPGs
can be activated by pharmacological treatment with
neurotransmitter agonists. In mammals, e.g. in cat
walking, this rhythmic activity has been called fictive
locomotion (Grillner 1981), because the coordination
of the various sets of motoneuron pools supplying the
leg joints bears similarities to their coordination
during in vivo stepping (Rybak et al 2005, Pear-
son 2008 ). Interestingly, the neural networks generat-
ing a basic motor output for stepping in vertebrates are
distributed across at least five segments in the lumbar
spinal cord, see also (Yakovenko et al 2002). The
notion of one single CPG controlling all motoneurons
and muscles supplying a limb of a mammal in
locomotion has been challenged for a long time: 30
years ago evidence from in vivo motor activity of
various cat preparations, e.g. (Grillner and Zang-
ger 1979) prompted (Grillner 1981) to question the
notion that there is one CPG for hind leg movements
in the cat lumbar spinal cord. In the light of the varying
coordination in muscle activity across leg joints the
6
Bioinspir. Biomim. 10 (2015) 041001 T Buschmann et al