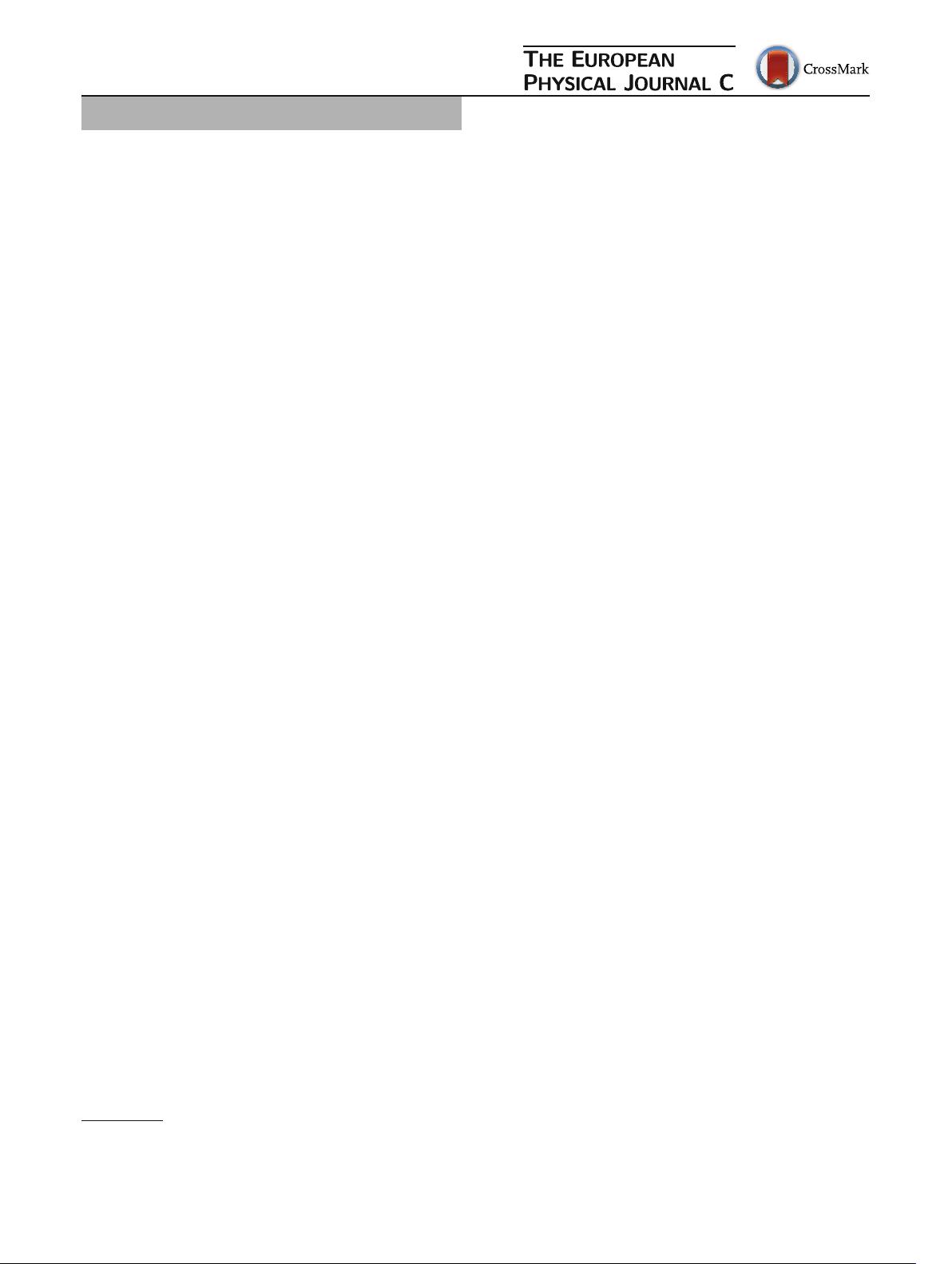
Eur. Phys. J. C (2017) 77:871
https://doi.org/10.1140/epjc/s10052-017-5413-5
Regular Article - Theoretical Physics
Stellar equilibrium configurations of white dwarfs in the f (R, T)
gravity
G. A. Carvalho
1,a
, R. V. Lobato
1,2,3
, P. H. R. S. Moraes
1
, José D. V. Arbañil
4
, E. Otoniel
5
,
R. M. Marinho Jr
1
, M. Malheiro
1
1
Departamento de Física, Instituto Tecnológico de Aeronáutica, São José dos Campos, SP 12228-900, Brazil
2
Dipartimento di Fisica, Sapienza Università di Roma, P.le Aldo Moro 5, 00185 Rome, Italy
3
ICRANet, P.zza della Repubblica 10, 65122 Pescara, Italy
4
Departamento de Ciencias, Universidad Privada del Norte, Avenida Alfredo Mendiola 6062 Urbanización Los Olivos, Lima, Peru
5
Instituto de Formação de Professores, Universidade Federal do Cariri, Brejo Santo, CE 63260-000, Brazil
Received: 9 October 2017 / Accepted: 23 November 2017 / Published online: 15 December 2017
© The Author(s) 2017. This article is an open access publication
Abstract In this work we investigate the equilibrium con-
figurations of white dwarfs in a modified gravity theory, na-
mely, f (R, T ) gravity, for which R and T stand for the Ricci
scalar and trace of the energy-momentum tensor, respec-
tively. Considering the functional form f (R, T ) = R +2λT ,
with λ being a constant, we obtain the hydrostatic equilibrium
equation for the theory. Some physical properties of white
dwarfs, such as: mass, radius, pressure and energy density,
as well as their dependence on the parameter λ are derived.
More massive and larger white dwarfs are found for negative
values of λ when it decreases. The equilibrium configurations
predict a maximum mass limit for white dwarfs slightly above
the Chandrasekhar limit, with larger radii and lower central
densities when compared to standard gravity outcomes. The
most important effect of f (R, T ) theory for massive white
dwarfs is the increase of the radius in comparison with GR
and also f (R) results. By comparing our results with some
observational data of massive white dwarfs we also find a
lower limit for λ, namely, λ>−3 ×10
−4
.
1 Introduction
White dwarfs (WDs) are the final evolution state of main
sequence stars with initial masses up to 8.5–10.6 M
.They
correspond to 95–97% of all observed stars in the Universe
[1]. The main sequence progenitors can reach sufficiently
high core temperatures (8–12×10
8
K), to proceed to carbon
burning and produce either oxygen-neon (ONe) core WDs or
undergo a core-collapse supernova (SNII) via electron cap-
ture on the products of carbon burning.
a
e-mail: araujogc@ita.br
Chandrasekhar has shown that a WD cannot sustain a mass
over 1.44M
, establishing the so-called Chandrasekhar mass
limit [2]. If a WD grows over this limit, as in binary systems
in which a WD is receiving mass from a nearby star, a type Ia
supernova (SNIa) explosion may occur. SNIa progenitors are
expectedto be similar, with nearly equal luminosity,therefore
being considered standard candles [3]. In fact, in the late
1990s, the use of SNIa led to the discovery that the expansion
of the Universe is accelerating [4,5].
Nevertheless, some super luminous SNIas were found
recently [6,7]. It has been suggested that their progenitors
are WDs that exceed the Chandrasekhar mass limit (2.1–2.8
M
·
)[6–11], being termed “super-Chandrasekhar WDs”.
WDs usually are not considered as a “laboratory” for
strong field regimes. However, general relativistic effects
have shownto be non-negligible in the massive and very mag-
netic WD regime [12–16]. Particularly, it has been shown in
[13] and [17] that the inclusion of general relativistic effects
tends to reduce the maximum mass of WDs with strong mag-
netic fields. Chandrasekhar and Tooper showed that instabil-
ity criteria for WDs under radial oscillations in a general
relativistic framework has its consequences for critical cen-
tral density depending on the composition of the star [18,19].
Moreover, in [20–22] it was shown that this critical central
density yields a larger minimum radius in comparison with
Newtonian gravity outcomes.
On this regard,despite the detection of gravitational waves
[23] and innumerable other positive results, as one can check,
for instance, in [24], there are two cosmological phenomena,
namely dark energy and dark matter, not well understood
within the General Relativity (GR) framework. Such a dark
sector of the universe composition is a possible indication
123