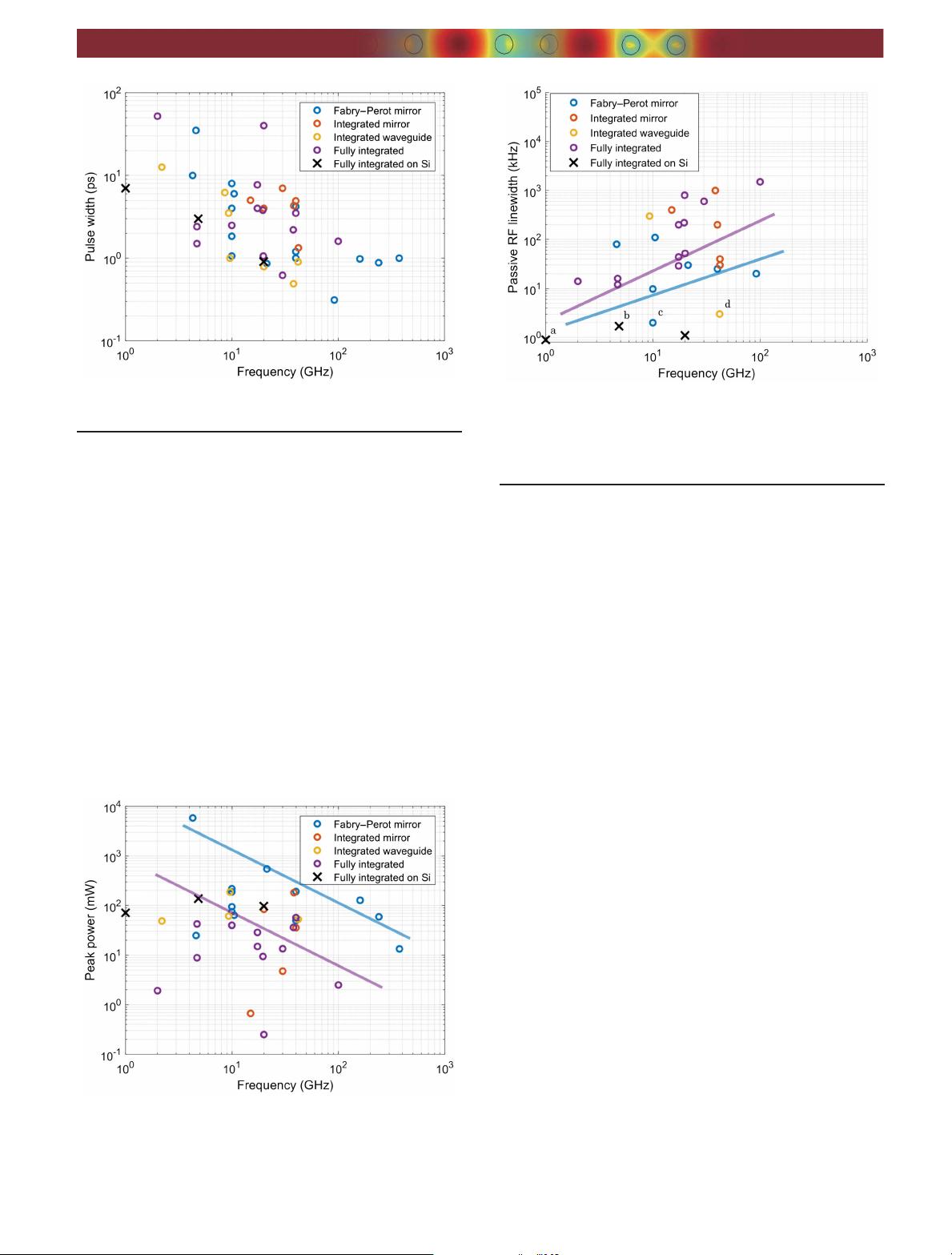
Figure 3 shows the peak power of lasers from the literature
survey. There is a generally decreasing trend of peak power ver-
sus repetition frequency, because the laser CW power is divided
up between more pulses at higher frequency. Integrated lasers
typically have 6–10 times lower peak power because of the
excess loss introduced by the integrated components. The
98 mW peak power of the laser presented in this work, shown
as a black “X”, is the highest of any fully integrated laser at
20 GHz. One integrated mirror laser, at 40 GHz, beats the
trend by integrating a high-power flared amplifier on-chip with
the laser [23]. Approximate trend lines are shown, representing
the decrease in peak power due to increasing repetition rate.
Fully integrated lasers are typically 1 order of magnitude lower
in peak power due to excess loss in the integrated components.
Figure 4 shows the 3 dB passively mode-locked RF line-
width of lasers from literature. There are comparatively fewer
data points on this chart, since this measurement is less com-
monly performed than autocorrelation. Like peak power, the
linewidth generally improves—trending downward—with
decreasing frequency. A few notable exceptions to the trend are
indicated with superscripts. “a” and “b”, like the laser presented
in this work, are heterogeneous silicon/InP lasers, specifically
with very long cavities formed from low-loss silicon wave-
guides, which reduces the Schawlow–Townes linewidth, a con-
tributor to the RF linewidth in mode-locked lasers [54]. The
laser at “a” reported only its 10 dB linewidth, which is shown in
the figure regardless; assuming a Lorentzian line shape, this
would correspond to 450 Hz 3 dB linewidth. The laser at “c”
uses the AlGaInAs quaternary for its waveguide and quantum
well, instead of the much more common InGaAsP. AlGaInAs is
reported to have lower linewidth enhancement factor than
InGaAsP [13,55], which should reduce the Schawlow–
Townes linewidth. The laser at “d” does not have a passive
waveguide; instead, it has a butt-joint-regrown uni-traveling-
carrier absorber design [30] and displays an impressive 900 fs
pulse width as well as the 3 kHz linewidth. Approximate trend
lines are shown, with blue representing Fabry–Perot lasers and
purple representing fully integrated lasers. The outliers at a, b,
c, and d were not considered part of the trend. It is notable that
linewidths of the three fully integrated heterogeneous Si lasers
are significantly lower than those of the majority of fully inte-
grated devices.
3. EXPERIMENT
The laser performance was optimized by producing a standard-
ized test laser in a high-yield fabrication process and then vary-
ing individual elements of the design. Thanks to wafer-scale
fabrication, many different device designs can be fabricated
Fig. 2. Pulse width of a selection of mode-locked laser diodes from
the literature.
Fig. 3. Peak power of a selection of lasers from the literature, in-
cluding this work. The colored lines represent approximate trends;
purple is for fully integrated lasers, and blue is for Fabry–Perot lasers.
The silicon lasers were not considered part of the trend.
Fig. 4. 3 dB passively-mode locked RF linewidth of a selection of
mode-locked lasers from the literature. The colored lines represent
approximate trends; purple is for fully integrated lasers, and blue is
for Fabry–Perot lasers. The silicon lasers and the two labeled outliers
were not considered part of the trend.
470 Vol. 6, No. 5 / May 2018 / Photonics Researc h
Research Article