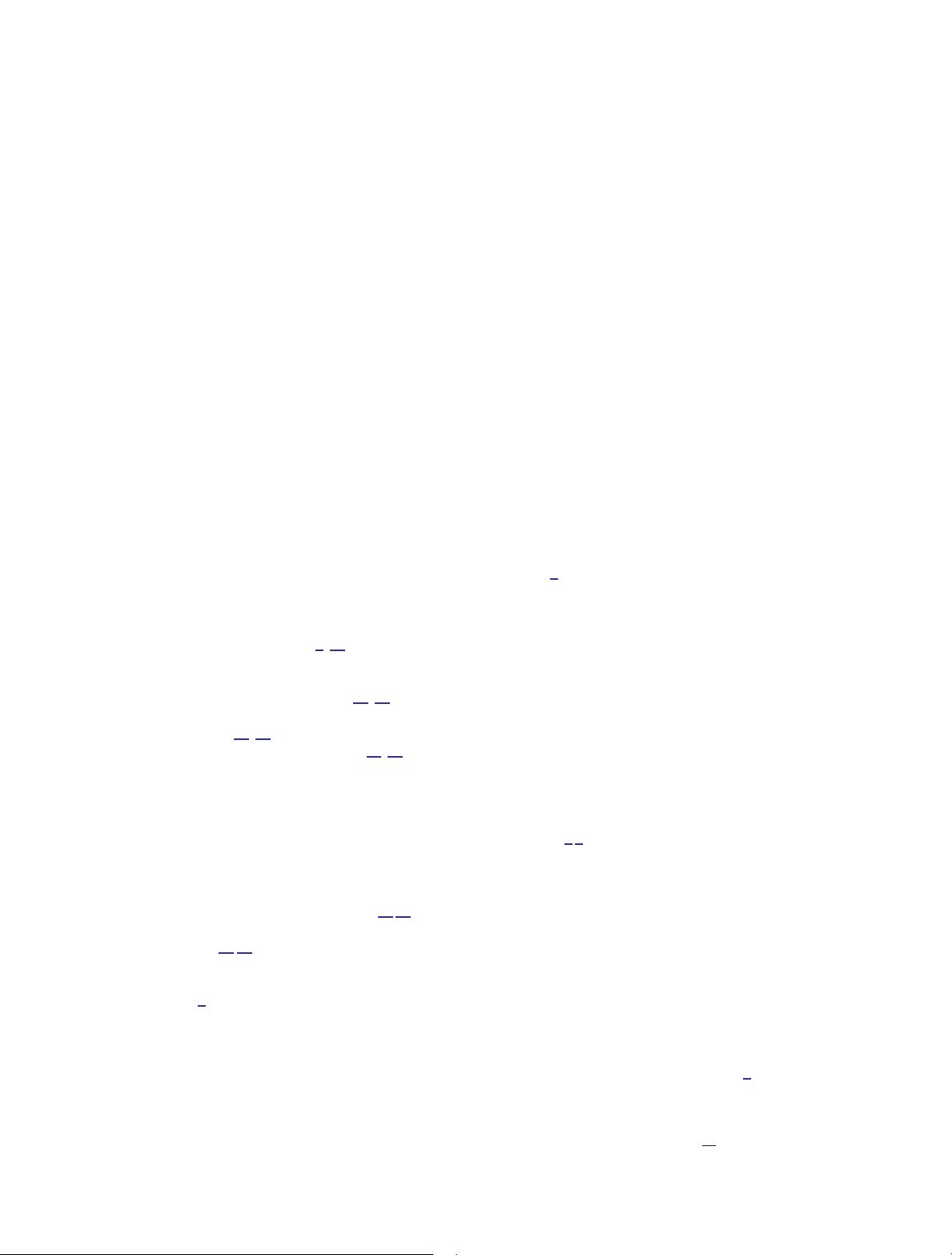
Ghost imaging for an axially moving target
with an unknown constant speed
Xiaohui Li, Chenjin Deng, Mingliang Chen, Wenlin Gong,* and Shensheng Han
Key Laboratory for Quantum Optics and Center for Cold Atom Physics, Shanghai Institute of Optics and Fine Mechanics,
Chinese Academy of Sciences, Shanghai 201800, China
*Corresponding author: gongwl@siom.ac.cn
Received March 25, 2015; revised April 29, 2015; accepted April 29, 2015;
posted May 5, 2015 (Doc. ID 236857); published June 12, 2015
The influence of the axial relative motion between the target and the source on ghost imaging (GI) is investigated.
Both the analytical and experimental results show that the transverse resolution of GI is reduced as the deviation of
the target’s center position from the optical axis or the axial motion range increases. To overcome the motion blur,
we propose a deblurring method based on speckle-resizing and speed retrieval, and we experimentally validate its
effectiveness for an axially moving target with an unknown constant speed. The results demonstrated here will be
very useful to forward-looking GI remote sensing. © 2015 Chinese Laser Press
OCIS codes: (110.2990) Image formation theory; (270.0270) Quantum optics; (110.1758) Computational
imaging; (100.2960) Image analysis.
http://dx.doi.org/10.1364/PRJ.3.000153
1. INTRODUCTION
Ghost imaging (GI), as a nonlocal imaging method, has theo-
retically and experimentally demonstrated that an unknown
target can be reconstructed without scanning the target, when
a single-pixel bucket detector is used to receive the target’s
transmitted (or reflected) signals [
1–10]. This technique
quickly aroused enormous interest in remote sensing, and fac-
tors affecting the imaging quality of GI, such as scattering and
turbulence, have been widely investigated [
11–20]. Recently,
super-resolution GI, three-dimensional GI lidar, and full-color
GI can also be achieved [
20–23]. However, all previous works
on GI have been concerned with static targets [
11–22]. In prac-
tical applications of remote sensing, imaging an unstable or
moving target is much more meaningful because there is usu-
ally relative motion between the imaging system and the target.
There are two cases of relative motion between the imaging
system and the target. One is in the tangential direction, and
the other is in the axial direction. For the former, some theo-
retical and experimental results have shown that the tangen-
tial relative motion between the GI system and the target will
cause the degradation of transverse resolution [
24,25]. Based
on the character of GI, some methods are invented to over-
come the motion blur [
26,27]. For the latter and its correspon-
dence to forward-looking imaging in practice, there is no
relevant discussion at present. According to the analytical re-
sults described in [
7], the magnification of recovered images
will vary with the axial distance between the source plane and
the target plane when the reference path of the GI system is
fixed. Therefore, the axial relative motion between the GI sys-
tem and the target will also lead to the degradation of trans-
verse resolution. In this work, the influence of axial motion on
GI is investigated both theoretically and experimentally. To
overcome the motion blur, a deblurring method for an axial
moving target with an unknown constant speed is invented
and its effectiveness is experimentally validated.
2. ANALYTICAL RESULTS
Figure 1 shows the experimental schematic of lensless
pseudo-thermal light GI for an axially moving target. The
pseudo-thermal light is obtained by passing a laser beam
through a slowly rotating ground glass disk (the wavelength
λ 532 nm, and the light spot’s transverse size D 2 mm on
the rotating ground glass disk plane). By using a beam splitter,
the light is divided into a test and a reference path. In the refer-
ence path, the light propagates directly to a charge-coupled
device (CCD) camera D
r
. In the test path, the intensity trans-
mitted through the target is collected by a lens f onto a single-
pixel bucket detector D
t
. The target is placed on a motion
platform, and the platform is driven by a stepping motor; thus
it can move along the optical axis. In addition, the stepping ac-
curacy of the platform is 1 μm, and the stepping motor is con-
nected to a computer, which can control the target’smotion.
For GI [
1,2], the second-order correlation function of inten-
sity fluctuations between two detectors is
ΔG
2;2
x
r
;x
t
Z
dx
1
Z
dx
2
G
1;1
x
1
;x
2
h
r
x
r
;x
1
h
t
x
t
;x
2
2
;
(1)
where G
1;1
x
1
;x
2
is the first-order correlation function of the
light field on the rotating ground glass plane. x
1
and x
2
are the
coordinates on the source plane. h
t
x
t
;x
2
is the impulse re-
sponse function of the test path, and h
r
x
r
;x
1
denotes the
phase conjugate of the impulse response function in the refer-
ence path. In addition, x
r
is the coordinate on the CCD camera
plane, and x
t
is the coordinate on the detector D
t
plane.
For the schematic shown in Fig.
1, under the paraxial
approximation, the impulse response function of the refer-
ence path is
h
r
x
r
;x
1
∝ exp
jπ
λz
x
r
− x
1
2
; (2)
Li et al. Vol. 3, No. 4 / August 2015 / Photon. Res. 153
2327-9125/15/040153-05 © 2015 Chinese Laser Press