没有合适的资源?快使用搜索试试~ 我知道了~
首页空间任务工程实用指南:微宇宙2005版
"Space Mission Analysis and Design-Microcosm (2005)" 是一本关于空间任务工程的实践手册,由顶尖的航空航天专家撰写,旨在引导读者了解从轨道选择到地面操作的整个任务设计过程。该书是SMAD(Space Mission Analysis and Design)系列的第三版,更新了技术内容,特别强调小型航天器设计和成本降低过程,并增加了多卫星制造、空间计算机、有效载荷设计以及自主系统等领域的详细信息。
在航天器任务分析和设计方面,这本书提供了深入的指导。对于地球静止卫星的任务设计,作者J.J.Poeba提供了一个入门介绍,涵盖了选择合适轨道的关键因素和考虑。而James R. Wertz和Wiley J. Larson合作的前两版SMAD书籍则为读者构建了坚实的基础,第三版在此基础上增加了新的视角和技术进步。
书中对小型航天器设计的强调反映了当前航天领域的一个趋势,即小型化和低成本化。随着技术的发展,小型卫星在科学研究、通信和地球观测等领域的作用日益显著。书中详细探讨了如何在有限的预算和尺寸限制下优化设计,以实现高效和可靠的太空任务。
关于多卫星制造,书中有专门的章节讨论如何管理和协调多个航天器的生产,这对于星座网络的构建至关重要。此外,空间计算机部分介绍了现代航天器上用于控制和处理任务数据的硬件,这些都是保证任务成功执行的核心组成部分。
在有效载荷设计方面,书中可能会涵盖传感器选择、数据传输和处理等主题,这些是航天器执行特定任务(如遥感或通信)的关键。自主系统部分则讨论了现代航天器如何通过自主导航、控制和决策系统来提高任务效率和生存能力。
降低成本是航天项目中的一个关键挑战,James R. Wertz和Wiley J. Larson的另一本著作专门探讨了这个话题,提供了策略和方法来减少航天任务的经济负担。这包括了从项目规划到实施的各个阶段的成本优化。
此外,书中的其他主题如空间结构和机械装置、空间飞行生命支持和生物圈学、逻辑微空间、空间营销、基础天体力学和应用、任务几何学、心理因素对产品开发的影响,以及空间飞行动力学和磁层的基本原理,都是全面理解航天任务设计不可或缺的知识点。它们共同构建了一套综合的学习资源,不仅适用于航天工程师,也适合于对此领域感兴趣的学者和学生。
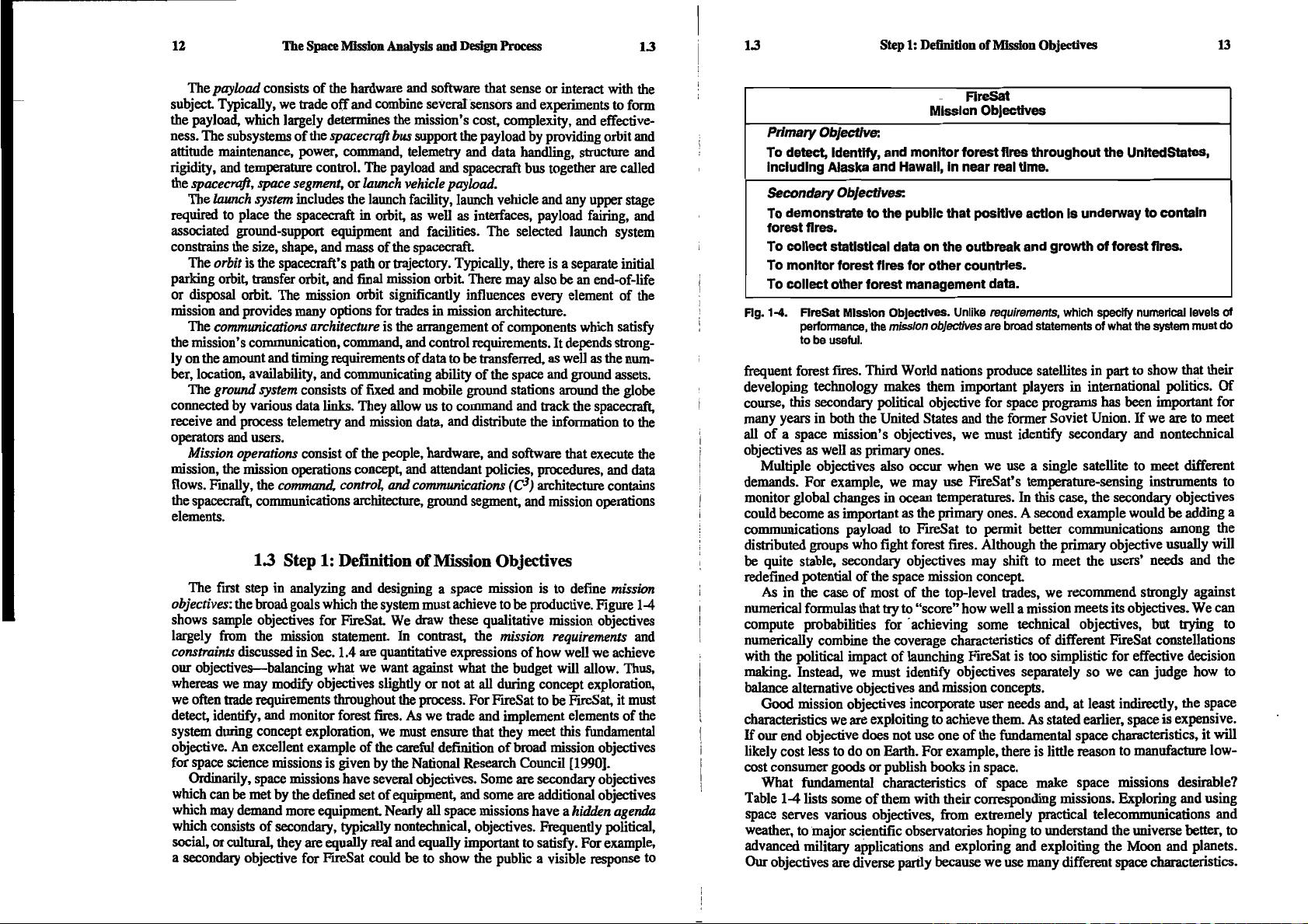
12
The
Space
Mission Analysis
and
Design
Process
1.3
The payload consists of the hardware
and
software that sense or interact
with
the
subject Typically,
we
trade off
and
combine
severaISensOrs
and
experiments to
form
the
payload,
which largely determines
the
mission's cost, complexity,
and
effective-
ness. The subsystems
of
the spacecraft bus support the payload by providing orbit
and
attitude maintenance, power,
co~d,
telemetry
and
data handling, structure
and
rigidity,
and
temperature control.
The
payload
and
spacecraft bus together are called
the
spacecraft, space segment, or launch vehicle payload.
The
launch system includes the launch facility, launch vehicle
and
any
upper
stage
required
to
place
the
spacecraft
in
orbit,
as
well
as
interfaces, payload fairing,
and
associated ground-support equipment
and
facilities. The selected launch
system
constrains
the
size,
shape,
and
mass
of
the
spacecraft.
The
orbit is
the
spacecraft's path or trajectory. Typically, there is a separate initial
parking orbit, transfer orbit,
and
final
mission orbit There
may
also
be
an
end-of-life
or
disposal
orbit The mission orbit significantly influences every element of
the
mission
and
provides
many
options
for
trades
in
mission architecture.
The
communications architecture is
the
arrangement
of
components
which
satisfy
the mission's communication,
command,
and
control requirements. It
depends
strong-
lyon
the
amount
and
timing requirements
of
data to be transferred,
as
well
as
the
num-
ber, location, availability,
and
communicating ability
of
the space
and
ground assets.
The
ground system consists of fixed
and
mobile ground stations
around
the globe
connected
by
various data links. They
allow
us
to command
and
track the spacecraft,
receive
and
process telemetry
and
mission data,
and
distribute the information to
the
operators
and
users.
Mission operations consist
of
the
people, hardware,
and
software that execute
the
mission,
the
mission operations concept,
and
attendant policies, procedures,
and
data
flows.
Fmally, the
command,
control, and communications (C
3
)
architecture contains
the
spacecraft, communications architecture, ground segment,
and
mission operations
elements.
1.3 Step 1: Definition
of
Mission Objectives
The first step
in
analyzing
and
designing a space mission is to define mission
objectives:
the broad goals
which
the
system
must achieve
to
be
productive. Figure
1-4
shows
sample
objectives
for
FrreSat
We
draw
these qualitative mission objectives
largely
from
the mission statement In contrast, the mission requirements
and
constraints discussed in Sec. 1.4
are
quantitative expressions
of
how
well
we
achieve
our objectives-balancing
what
we
want
against what the budget will
allow.
Thus,
whereas
we
may
modify
objectives slightly or not at
all
during concept exploration,
we
often
trade
requirements throughout
the
process. For FrreSat to be FrreSat, it
must
detect, identify,
and
monitor forest fires.
As
we
trade
and
implement elements
of
the
system during concept exploration,
we
must ensure that they meet this
fundamental
objective. An excellent example
of
the careful definition
of
broad mission objectives
for space science missions is given by
the
National Research Council [1990].
Ordinarily, space missions have several objectives. Some are secondary objectives
which
can
be
met by the defined set
of
equipment,
and
some are additional objectives
which
may
demand
more equipment Nearly
all
space missions have a hidden
agerula
which consists
of
secondary, typically nontechnical, objectives. Frequently political,
social, or cultural, they
are
equally real
and
equally important to satisfy. For
example,
a secondary objective for FrreSat could
be
to
show
the public a visible response
to
1.3
Step
1:
Definition
of
Mission Objectives
13
-
RreSat
Mission Objectives
Primary
ObJectJve:
To detect, Identify, and monitor forest fires throughout the UnltedStates,
Including Alaska and HawaII, In near real time.
Secondary ObJectives:
To demonstrate
to
the public that positive action
Is
underway
to
contain
forest fires.
To collect statistical data on the outbreak and growth
of
forest fires.
To monitor forest fires
for
other countries.
To collect other forest management data.
Fig. 1-4. FlreSat
Mission
ObJectives. Unlike requirements, which specify numerical levels
of
performance,
the mission objectives are
broad
statements of what the system must do
to
be
useful.
frequent forest fires. Third World nations produce satellites in part to
show
that their
developing technology
makes
them important players
in
international politics.
Of
course, this secondary political objective for space programs has been important for
many
years
in
both the United States
and
the former Soviet Union.
If
we
are to meet
all
of
a space mission's objectives,
we
must identify secondary
and
nontechnical
objectives
as
well
as
primary ones.
Multiple objectives also occur
when
we
use a single satellite to meet different
demands. For example,
we
may
use FIreSat's temperature-sensing instruments to
monitor global changes
in
ocean temperatures. In this case, the secondary objectives
could become as important
as
the primary ones. A second example would
be
adding a
communications payload
to FrreSat to permit better communications among the
distributed groups
who
fight forest fires. Although the primary objective usually will
be
quite stable, secondary objectives may shift
to
meet the users' needs and the
redefined potential of the space mission concept
As
in
the
case
of
most of the top-level trades,
we
recommend strongly against
numerical formulas that try to "score"
how
well a mission meets its objectives.
We
can
compute probabilities
for'
achieving some technical objectives, but trying to
numerically combine the coverage characteristics
of
different FrreSat constellations
with the political impact
of
launching FrreSat is too simplistic for effective decision
making. Instead,
we
must identify objectives separately so
we
can judge how to
balance alternative objectives and mission concepts.
Good mission objectives incorporate user needs and, at least indirectIy, the space
characteristics
we
are exploiting to achieve them.
As
stated earlier, space is expensive.
If
our end objective does not use one
of
the fundamental space characteristics, it
will
likely cost less to do
on
Earth. For example, there is little reason to manufacture low-
cost consumer goods or publish
books in space.
What fundamental characteristics
of
space make space missions desirable?
Table 1-4 lists some
of
them with their corresponding missions. Exploring and using
space serves various objectives, from extremely practical telecommunications and
weather, to major scientific observatories hoping
to understand the universe better, to
advanced military applications and exploring
and
exploiting the Moon and planets.
Our objectives are diverse partIy because we use many different space characteristics.
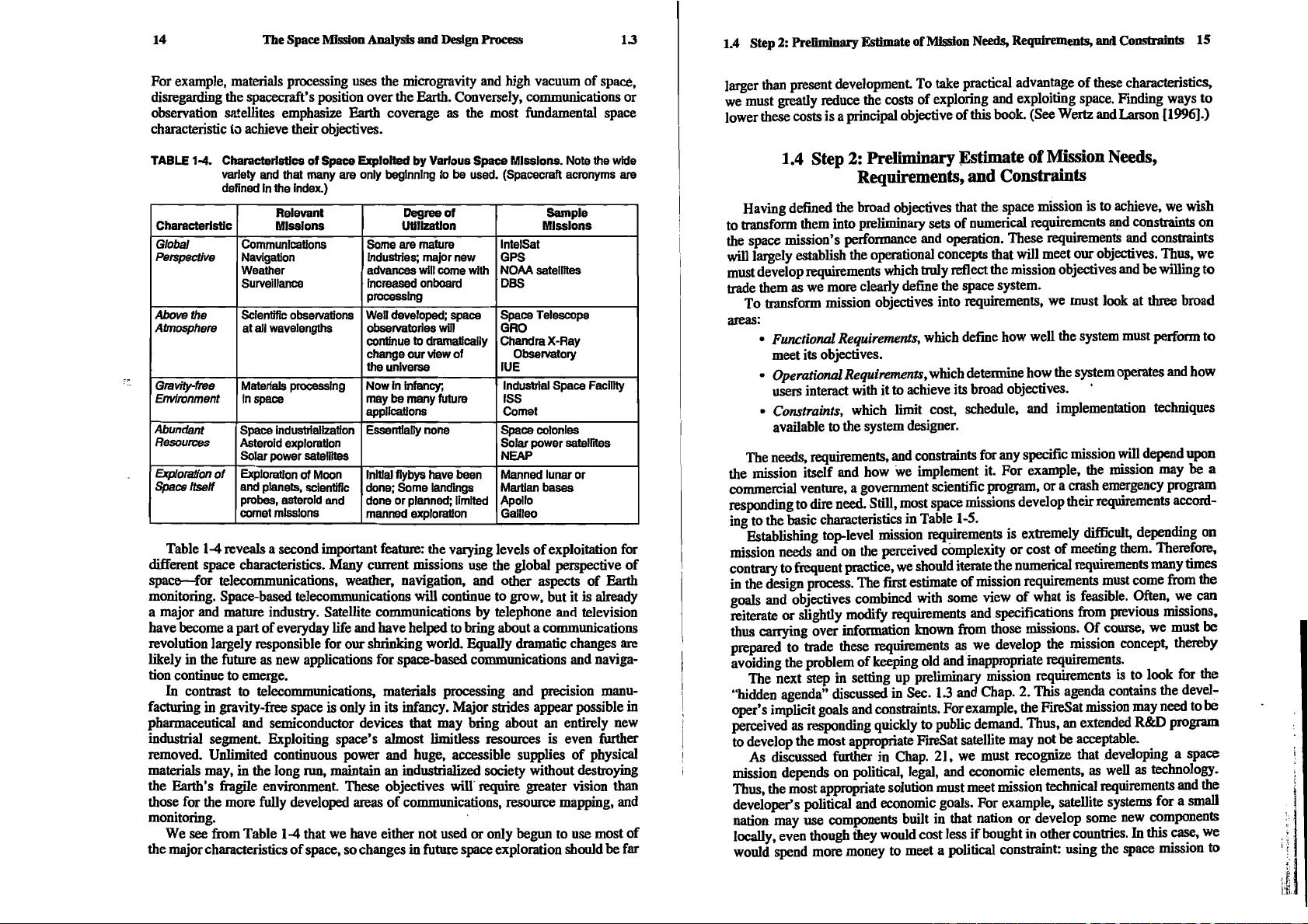
14
The
Space Mission Analysis and Design Process
1.3
For example, materials processing uses the microgravity and high vacuum of space,
disregarding the spacecraft's position over the Earth. Conversely, communications or
observation satellites emphasize Earth coverage
as
the most fundamental space
characteristic to achieve their objectives.
TABLE 1-4. Characteristics
of
Space Exploited by Various Space Missions.
Note
the
wide
variety
and
that many are only beginning to
be
used. (Spacecraft
acronyms
are
defined
In
the
Index.)
Relevant
Degree
of
Sample
CharacterisUc
Missions
UtIlization Missions
Global Communications
Some
are
mature
IntelSat
Perspective
Navigation Industries; major new
GPS
Weather advances will
corne
with
NOAA
satellites
Surveillance Increasad
onboard
DBS
processing
Above
the
Scientific observations
WeD
developed;
space
Space Telescope
Atmosphere at all wavelengths observatories
will
GAO
continue to
dramatlcaDy
Chandra X-Ray
change our view of Observatory
the unlversa
IUE
Gravity-free
MaterIaJs
processing
Now
In
Infancy; Industrial Space Facility
EnvIronment
In
space
may
be many future
ISS
applications
Cornet
Abundant
Space industrialization
Essentially
none
Space colonies
Resources
Asteroid exploration Solar power
satemtes
Solar power
sateDites
NEAP
Exploration
of
Exploration of
Moon
Initial flybys have been
Manned lunar
or
Space Itself
and
planets, scientific
done;
Some
landings Martian basas
probes, asteroid
and
done
or planned; limited Apollo
comet missions
manned
exploration Gailleo
Table 1-4 reveals a second
impOrtant
feature: the varying levels
of
exploitation for
different space characteristics. Many current missions use the global perspective
of
space--for telecommunications, weather, navigation, and other aspects
of
Earth
monitoring. Space-based telecommunications
will
continue to grow, but it is already
a major and mature industry. Satellite communications by telephone and television
have become a part
of
everyday life and have helped to bring about a communications
revolution largely responsible for our sbrinking world. Equally dramatic changes
are
likely in the future as new applications for space-based communications and naviga-
tion continue to emerge.
In
contrast to telecommunications, materials processing and precision manu-
facturing in gravity-free space
is only in its infancy. Major strides appear possible in
pharmaceutical and semiconductor devices
that may bring about an entirely new
industrial segment Exploiting space's almost limitless resources is even further
removed. Unlimited continuous power and huge, accessible supplies
of
physical
materials may, in the long run, maintain
an
industrialized society without destroying
the Earth's fragile environment These objectives will"
require
greater vision than
those for the more fully developed areas
of
communications, resource mapping,
and
monitoring.
We
see from Table 1-4 that
we
have either not used or only begun to use most
of
the
ouUor
characteristics
of
space, so changes in future space exploration should be far
1.4 Step 2: PreUminary Estimate
of
Mission Needs, Requirements, and Constraints 15
larger
than
present development To take practical advantage
of
these characteristics,
we
must greatly reduce the costs
of
exploring and exploiting space. Fmding ways
to
lower these costs is a principal objective
of
this book. (See Wertz and Larson [1996].)
1.4 Step 2: Preliminary J£stjmate
of
Mission Needs,
Requirements,
and
Constraints
Having defined the broad objectives that the space mission is to achieve, we wish
to transfonn them into preliminary sets
of
numerical requirements and constraints on
the space mission's performance and operation. These requirements and
constraints
will
largely establish the operational concepts that will meet our objectives. Thus, we
must develop requirements which truly reflect the mission objectives and be willing to
trade them
as
we
more clearly define the space system.
To transfonn mission objectives into requirements, we must look at
three broad
areas:
• Functional Requirements, which define how well the system must perfonn to
meet its objectives.
• Operational Requirements, which determine how the system operates
and
how
users interact with it
to
achieve its broad objectives. '
• Constraints, which limit cost, schedule, and implementation techniques
available to the system designer.
The needs, requirements, and constraints for any specific mission will depend upon
the mission itself and how
we
implement it. For example, the mission may be a
commercial venture, a government scientific program, or a crash emergency program
responding to dire need. Still, most space missions develop their requirements accord-
ing to the basic characteristics in Table 1-5.
Establishing top-level mission requirements is extremely difficult, depending on
mission needs and on the perceived complexity or cost of meeting them. Therefore,
contrary to frequent practice,
we
should iterate the numerical requirements many times
in the design process. The first estimate
of
mission requirements must come from the
goals and objectives combined with some view
o.f
wh~t
is feasible.
~n,
~e.
can
reiterate or slightly modify requirements and specifications from
preVIOUS
JD1SSlOns.
thus carrying over information known from those missions ..
Of.
course, we must be
prepared to trade these requirements
as
we
develop the
lDlSS10n
concept, thereby
avoiding the problem
of
keeping old and inappropriate requirements.
The next step in setting up preliminary mission requirements
is
to look for the
"hidden agenda" discussed in Sec.
1.3
and Chap. 2. This agenda contains the
devel~
oper's implicit goals and constraints. For example, the FrreSat mission may need to be
perceived
as
responding quickly to public demand. Thus,
an
extended R&D program
to develop the most appropriate FrreSat satellite may not be acceptable.
As discussed further in Chap. 21, we must recognize that developing a space
mission depends on political, legal, and economic elements,
as
well
as
technology.
Thus, the most appropriate solution must meet mission
technic~
requirements and the
developer's political and economic goals. For example, satellIte systems for a small
nation may use components built in that nation or develop some
new
components
locally, even though ihey would cost
less.i~bought
in
'?therc~untries.
In
this~,
we
would spend more money to meet a polItical constraInt: usmg the space
lDlSSlon
to
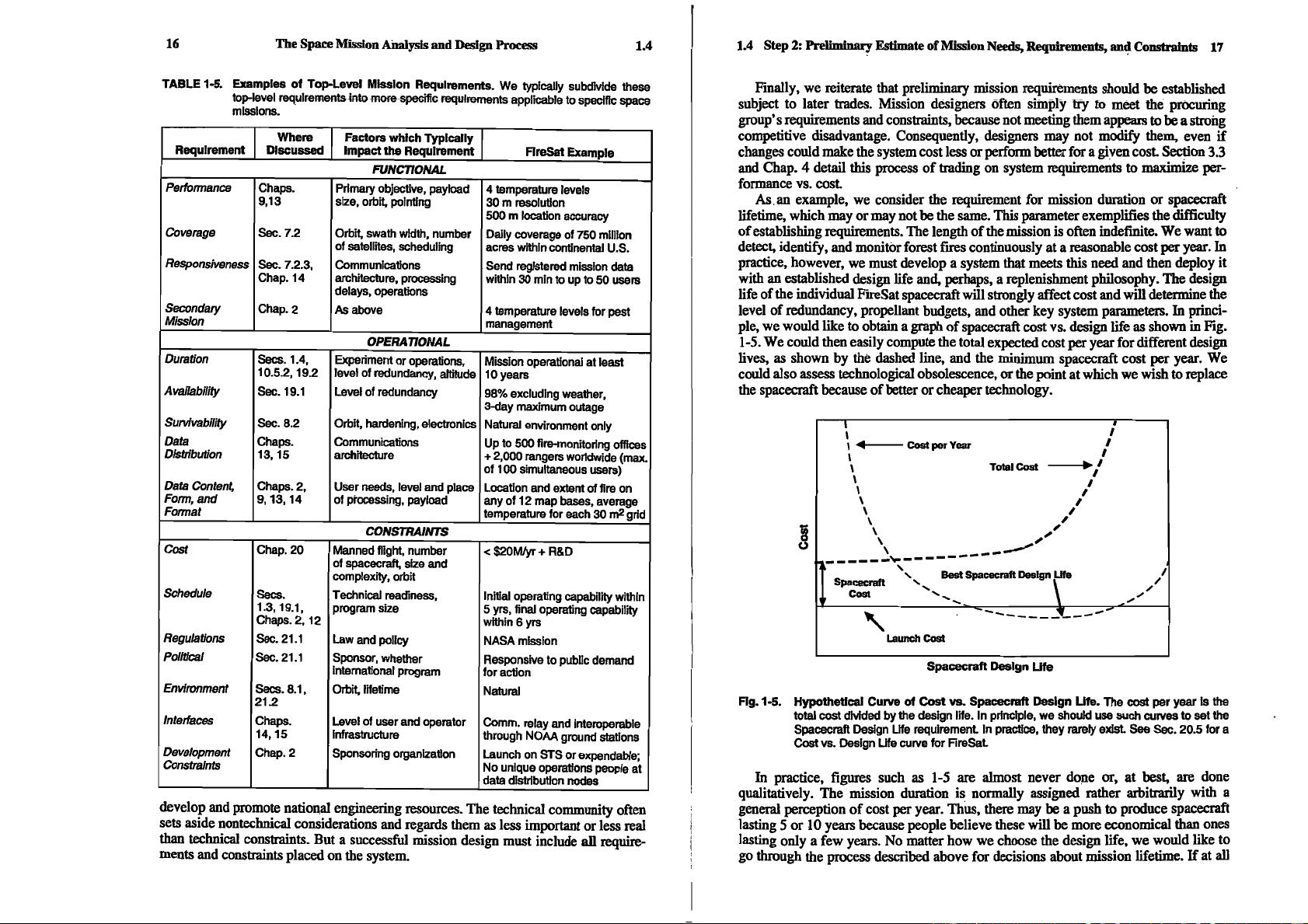
------------------------------------~~---~--------
16
The
Space Mission AiJalysis
and
Design Process
1.4
TABLE
1-5. Examples
of
Top-Level
Mission
Requirements. We typically subdivide these
top-level
requlrements~Rtomore~c
requirements applicable
to
specific space
missions.
Where
Fectors
which
Typically
Requirement
Discussed
Impact
the
Requirement
RreSat
Example
FUNCTIONAL
Performance
Chaps.
Primary objective. paytoad
4 temperature levels
9.13
size. orbit, pointing
30
m resolution
500 m location accuracy
Coverage
Sec. 7.2
Orbit, swath
width. number
Daily coverage of 750 million
of satelrltes. scheduling
acres within continental U.S.
Responsiveness
Sec. 7.2.3.
Communications
Send registered mission data
Chap.
14
architecture, processing
within
30
min to up
to
50 users
delays. operations
Secondary
Chap. 2
As above
4 temparature levels for
pest
Mission
management
OPERAnONAL
Duration
Sees. 1.4,
Experiment
or
operations,
Mission operational at least
10.5.2. 19.2
levelofredundancy.ahftuda
10 years
Availability
Sec.
19.1
Level of redundancy
98% excluding weather,
3-day maximum outage
Survivability
Sec. 8.2
Orbit, hardening. electronics
Natural environment only
Data
Chaps.
Communications
Up to 500 fire-monitoring offices
Distribution
13.15
architecture
+ 2.000 rangers worldwide (max.
of
100 simultaneous users)
Data Content, Chaps. 2,
User
needs. level and place
Location and extent
of fire on
Form,and
9.13,14
of
processing. payload
any
of
12
map
bases.
average
Format
temperature for each 30 m
2
grid
CONSTRAINTS
Cost
Chap.
20
Manned flight, number
<
$2OM/yr
+ R&D
of spacecraft, size and
complexity. orbit
Schedule
Sees.
Technical readiness.
Initial operating capability within
1.3,19.1.
program size
5
yrs. final operating capability
Chaps.
2,
12
within
6yrs
Regulations
Sec.
21.1
Law and policy
NASA mission
Political
Sec.
21.1
Sponsor. whether
Responsive to public demand
international program
for action
Environment
Sees. 8.1.
Orbit, lifetime
Natural
21.2
Interfaces
Chaps.
Level of user and operator
Comm. relay and Interoperable
14.15
Infrastructure
through NOAA ground s1ations
Development
Chap. 2
Sponsoring organization
Launch on STS
or
expendable;
Constraints
No
unique operations people at
data distribution nodes
develop and promote
natio~al
engineering resources. The technical community often
sets aside nontechnical considerations and regards them
as
less important or less real
than technical constraints. But a successful mission design must include
all require-
ments and constraints placed on the system.
1.4 Step 2: Preliminary Estimate
of
Mission Needs, Requirements,
an~
Constraints 17
Finally, we reiterate that preliminary mission requirements should
be
established
subject to later trades. Mission designers often . simply
lty
to meet the procuring
group's requirements and constraints, because not meeting them appears
to
be
a strong
competitive disadvantage. Consequently, designers may not modify them, even
if
changes could make the system cost less or perform better for a given
cost
Section
3.3
and Chap. 4 detail this
process
of
trading on system requirements to maximize per-
formance
vs.
cost
As.
an
example, we consider
the
requirement for mission duration or spacecraft
lifetime, which mayor may not
be
the same. This parameter exemplifies the difficulty
of
establishing requirements. The length
of
the mission is often indefinite. We want to
detect, identify, and monitor forest
fIreS
continuously at a reasonable cost per year.
In
practice, however,
we
must develop a system
that
meets this need and then deploy it
with an established design life and, perhaps, a replenishment philosophy.
The
design
life of the individual FtreSat
spacecraft
will strongly affect cost and will determine
the
level
of
redundancy, propellant budgets, and other key system parameters.
In
princi-
ple, we would like to obtain a graph
of
spacecraft cost vs. design life
as
shown in Fig.
1-5. We could then easily compute the total expected cost per year for different design
lives, as shown by the dashed line, and the minimum spacecraft cost per year.
We
could also assess technological obsolescence, or
the
point at which we wish to replace
the spacecraft because
of
better or cheaper technology.
\ ;
\
+---
Cost per
Year
"
\
\ Total Cost
~
I
\ I
\ "
\ ,
\ ,
\ ,
\ '
\ "
-----~.---------~
"
" Best
Spacecraft
DesignS
life
Spacecraft
"
....
Cost
.......
,
......
,
-------""----
Launch Cost
Spacecraft
Design
life
Rg.1-5.
HypotheUcai Curve
of
Cost
vs. Spacecraft Design
life.
The cost per year Is the
total
cost dMded by the design life. In principle,
we
should use such curves to
set
the
Spacecraft DesIgn
life
requirement In practice, they rarely exist.
See
Sec. 20.5 for a
Cost
vs. DesIgn
life
curve for
FireSat
In practice, figures such as
1-5
are almost never done or, at best, are done
qualitatively. The mission duration is normally assigned rather arbitrarily with a
general perception
of
cost per year.
ThUs,
there may be a push to produce spacecraft
lasting 5 or
10
years because people believe these will be more economical than ones
lasting only a few years. No matter how we choose the design life, we would like to
go through the process described above for decisions about mission lifetime.
If
at
all
'11
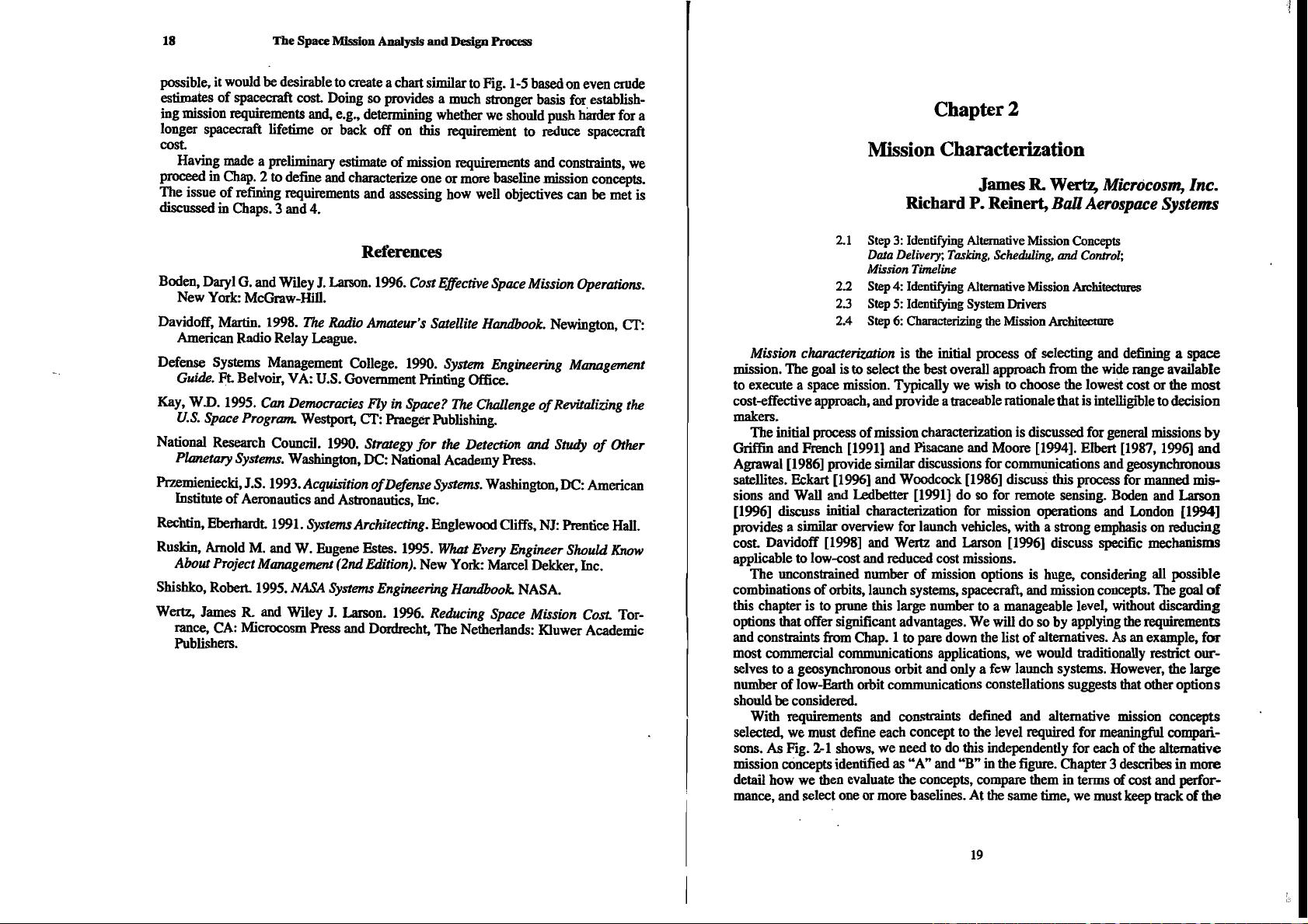
18
The
Space
Mission Analysis
and
Design Process
possible, it would be
desirable.
to create a chart similar to Fig.
1-5
based on even crude
estimates
of
spacecraft
cost
Doing so provides a much stronger basis
for,
establish-
ing mission requirements
and,
e.g., determining whether
we
should push harder for a
longer spacecraft lifetime or back off on
this requirement to reduce spacecraft
cost
Having made a
preliminary
estimate of mission requirements and constraints,
we
proceed
in Chap. 2 to define
and
characterize one or more baseline mission concepts.
The issue
of
refining requirements and assessing how well objectives can be met is
discussed in Chaps. 3 and 4.
References
Boden, Daryl
G.
and WIley
1.
Larson.
1996. Cost Effective Space Mission Operations.
New York: McGraw-Hill.
Davidoff, Martin. 1998.
The Radio Amateur's Satellite Handbook. Newington, CI':
American Radio Relay League.
Defense Systems Management College. 1990.
System Engineering Management
Guide.
Ft
Belvoir,
VA:
U.S. Government Printing Office.
Kay,
WD.
1995. Can Democracies Fly in Space? The Challenge
of
Revitalizing the
U.S. Space Program.
Westport, CI': Praeger Publishing.
National Research Council. 1990.
Strategy
for
the
Detection
and
Study
of
Other
Planetary Systems.
Washington, DC: National Academy Press.
Przemieniecki, J.S. 1993.
Acquisition
of
Defense Systems. Washington, DC: American
Institute
of
Aeronautics and Astronautics, Inc.
Rechtin, Eberhardt 1991.
Systems Architecting. Englewood Cliffs,
NJ:
Prentice Hall.
Ruskin, Arnold
M.
and
W.
Eugene Estes. 1995. What Every Engineer Should
Know
About Project Management (2nd Edition). New York: Marcel Dekker,lnc.
Shisbko, Robert 1995.
NASA Systems Engineering Handbook. NASA.
Wertz, James
R.
and WIley J. Larson. 1996. Reducing Space Mission Cost. Tor-
rance,
CA:
Microcosm Press and Dordrecht, The Netherlands: Kluwer Academic
Publishers.
Chapter 2
Mission Characterization
James R. Wert7,
Microcosm,
Inc.
Richard P. Reinert,
BaD
Aerospace
Systems
2.1 Step 3: Identifying Alternative Mission Concepts
Data
Delivery;
Tasking,
Scheduling,
and
Control;
Mission
Timeline
2.2 Step 4: Identifying Alternative Mission Architectures
2.3 Step 5: Identifying System Drivers
2.4 Step 6: Characterizing the Mission Architecture
Mission characterization is the initial process of selecting and defining a space
mission. The goal is
to select the best overall approach from the
wide
range available
to execute a space mission. Typically we
wish
to choose the
loweSt
cost or the most
cost-effective approach, and provide a traceable rationale that is intelligtble to decision
makers.
The initial process
of
mission characterization is discussed for general missions
by
Griffin and French [1991] and Pisacane and Moore [1994]. Elbert
[1987,
1996]
and
Agrawal [1986] provide similar discussions for communications
and
geosynchrono1JS
satellites. Eckart [1996] and Woodcock [1986] discuss this process for manned mis-
sions and Wall
and
Ledbetter [1991] do so for remote sensing.
Boden
and Larson
[1996] discuss initial characterization for mission operations and London [1994]
provides a similar overview for launch vehicles, with a strong emphasis on reducing
cost. Davidoff [1998] and Wertz and Larson [1996] discuss specific
mechanisms
applicable to low-cost and reduced cost missions.
The unconstrained number
of
mission options is huge, considering all possible
combinations of orbits, launch systems, spacecraft, and mission concepts. The goal
of
this chapter
is
to prune this large number to a manageable level, without discarding
options that offer significant advantages. We will do so by applying the requirements
and constraints from Chap. 1
to pare
down
the list
of
alternatives.
As
an
example, for
most commercial communications applications, we would traditionally restrict our-
selves to a geosynchronous orbit and only a
few
launch systems. However, the large
number
of
low-Earth orbit communications constellations suggests that other options
should be considered.
With requirements and constraints defined and alternative mission concepts
selected, we must define each concept to the level required for meaningful compari-
sons. As Fig.
2-1
shows,
we
need to
do
this independently for each of the alternative
mission
cOncepts
identified
as
"A" and "B" in the figure. Chapter 3
describes
in more
detail how
we
then evaluate the concepts, compare them in terms of cost and perfor-
mance, and select one or more baselines. At
the
same time, we must keep track
of
the
19
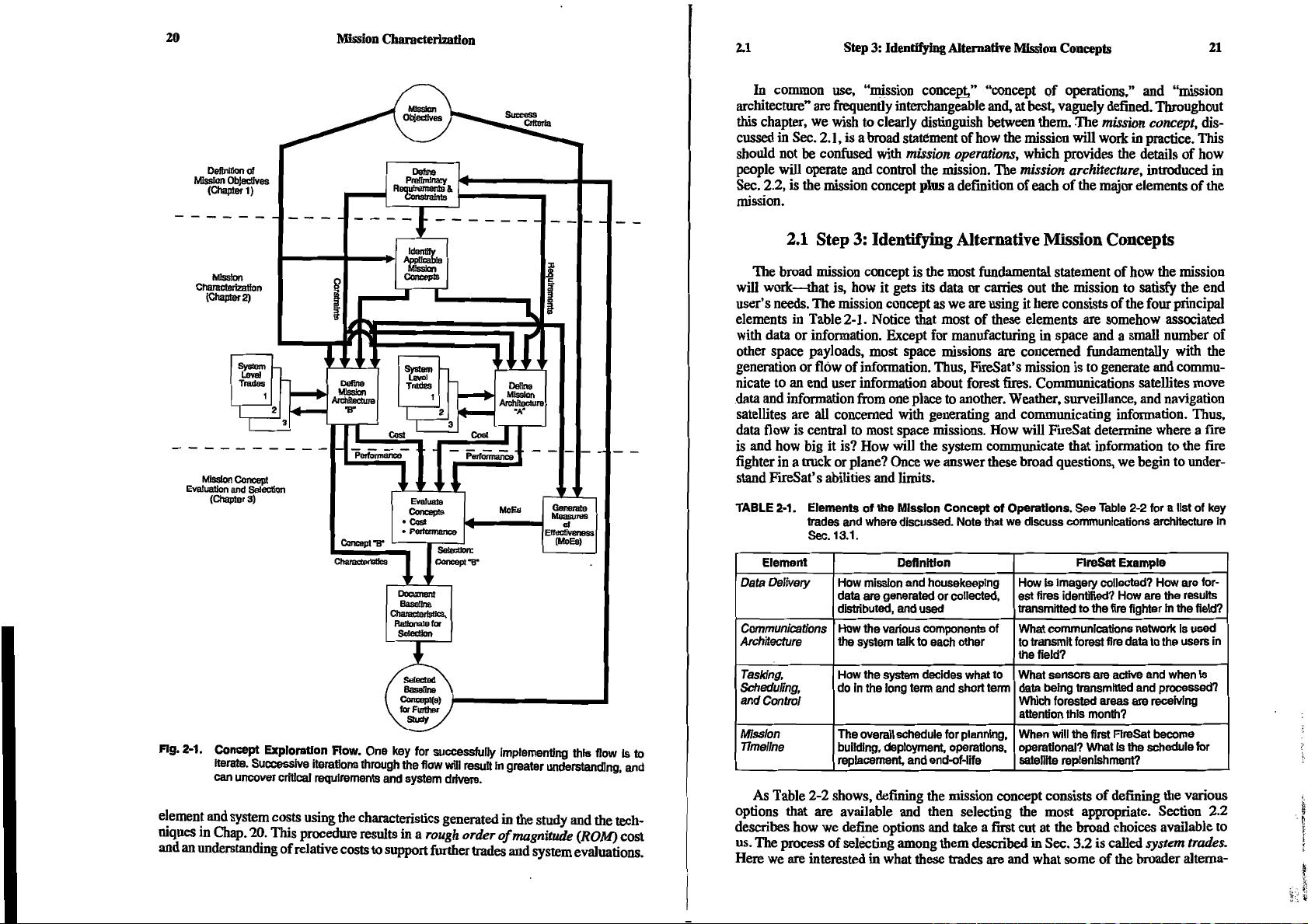
20
DeIInIIIon
of
MIssIon
Objecllves
(Chapter 1)
Mlss!on
CharacIerlzat!on
(Chapter 2)
MlssIon
Concept
Evaluation
and
Selection
(Chapter
3)
Mission Characterization
Evaluate
Concepts
• Cost
• Perlarmance
MoEs
~
Measures
01
EIfec!Iveness
(MoEs)
Fig. 2-1. Concept Exploration Flow.
One
key for successrully implementing this flow is to
iterate. Successive iterations through
the
flow
will
result in greater understanding and
can
uncover critical requirements
and
system drivers. '
e~emen~
and
system
c~ts
using the characteristics generated
in
the
study and the tech-
ruques
In
Chap.
20.
This procedure results
in
a rough order
of
magnitude (ROM) cost
and
an
understanding
of
relative costs to support further trades
and
system evaluations.
2.1
Step
3:
Identifying Alternative Mission Concepts
21
In
common
use,
"mission concept" "concept
of
operations:'
and
"mission
architecture"
are
frequently interchangeable
and,
at best, vaguely defined. Throughout
this
chapter,
we
wish to clearly distinguish between them. The mission concept, dis-
cussed in
Sec. 2.1, is a broad statement
of
how
the
mission will
wOIk
in
practice. This
should not
be
confused with mission operations, which provides the details
of
how
people
will operate
and
control
the
mission. The mission architecture, introduced
in
Sec. 2.2, is the mission concept plus a definition
of
each
of
the major elements
of
the
mission.
2.1 Step 3: Identifying Alternative Mission Concepts
The broad mission concept is
the
most fundamental statement
of
how
the mission
will
work-that
is,
how
it gets its data or carries out the mission to satisfy the end
user's needs. The mission concept
as
we
are
using it here consists of the four principal
elements in Table 2-1. Notice that most
of
these elements are somehow associated
with
data or infonnation. Except for manufacturing in space
and
a small number
of
other space payloads, most space missions are concerned fundamentally with
the
generation or
flow
of infonnation. Thus, FrreSat's mission is to generate
and
commu-
nicate to
an
end user infonnation about forest fires. Communications satellites
move
.data
and
information
from
one
place
to
another. Weather, surveillance,
and
navigation
satellites are
all
concerned with generating
and
communicating information. Thus,
data
flow
is
centmI
to
most
space missions.
How
will FireSat determine where a lIfe
is
and
how
big it is?
How
will
the
system communicate that information to
the
fire
fighter
in
a truck or plane?
Once
we
answer these broad questions,
we
begin to under-
stand FrreSat's abilities
and
limits.
TABLE 2-1. Elements
of
the
Mission Concept
of
Operations. See
Table
2-2 for a list of
key
trades and where discussed. Note that
we
discuss communications architecture in
Sec. 13.1.
Element
Definition
FlreSat Example
Data Delivery
How mission
and
housekeeping
How is imagery collected? How are for-
data are generated or collected,
est
fires identified? How are the results
distributed,
and
used
transmitted to the fire fighter in the field?
Communications
How
the various components of
What
communications network is
used
Architecture
the system talk to each other
to transmit forest fire data to the users in
the field?
Tasking,
How
the system decides what to
What sensors are
active
and
when is
Scheduling,
do
In
the long term and short term
data being transmitted
and
processed?
and
Control
WhIch forested areas are receMng
attention this month?
Mission
The overall schedule for planning,
When will
the first
F1reSat
become
Timeline
building, deployment, operations,
operational? What is the schedule for
replacement,
and
end-of-life
sateDHe
replenishment?
As
Table 2-2 shows, defining the mission concept consists
of
defining the various
options that are available
and
then
selecting the most appropriate. Section 2.2
describes how
we
define options
and
take a first cut at the broad choices available to
us.
The process
of
selecting among them described in Sec. 3.2 is called system trades.
Here
we
are interested in what these trades are and what some
of
the broader alterna-
剩余503页未读,继续阅读
2022-06-21 上传
2023-07-02 上传
2022-03-06 上传
2022-01-28 上传
2022-01-13 上传
2022-01-13 上传
2022-02-10 上传

zhanghc526
- 粉丝: 0
上传资源 快速赚钱
我的内容管理 展开
我的资源 快来上传第一个资源
我的收益
登录查看自己的收益我的积分 登录查看自己的积分
我的C币 登录后查看C币余额
我的收藏
我的下载
下载帮助

最新资源
- 支付宝订单监控免签工具:实时监控与信息通知
- 一键永久删除QQ空间说说的绿色软件
- Appleseeds训练营第4周JavaScript练习
- 免费HTML转CHM工具:将网页文档化简成章
- 奇热剧集站SEO优化模板下载
- Python xlrd库:实用指南与Excel文件读取
- Genegraph:通过GraphQL API使用Apache Jena展示RDF基因数据
- CRRedist2008与CRRedist2005压缩包文件对比分析
- SDB交流伺服驱动系统选型指南与性能解析
- Android平台简易PDF阅读器的实现与应用
- Mybatis实现数据库物理分页的插件源码解析
- Docker Swarm实例解析与操作指南
- iOS平台GTMBase64文件的使用及解密
- 实现jQuery自定义右键菜单的代码示例
- PDF处理必备:掌握pdfbox与fontbox jar包
- Java推箱子游戏完整源代码分享
安全验证
文档复制为VIP权益,开通VIP直接复制
