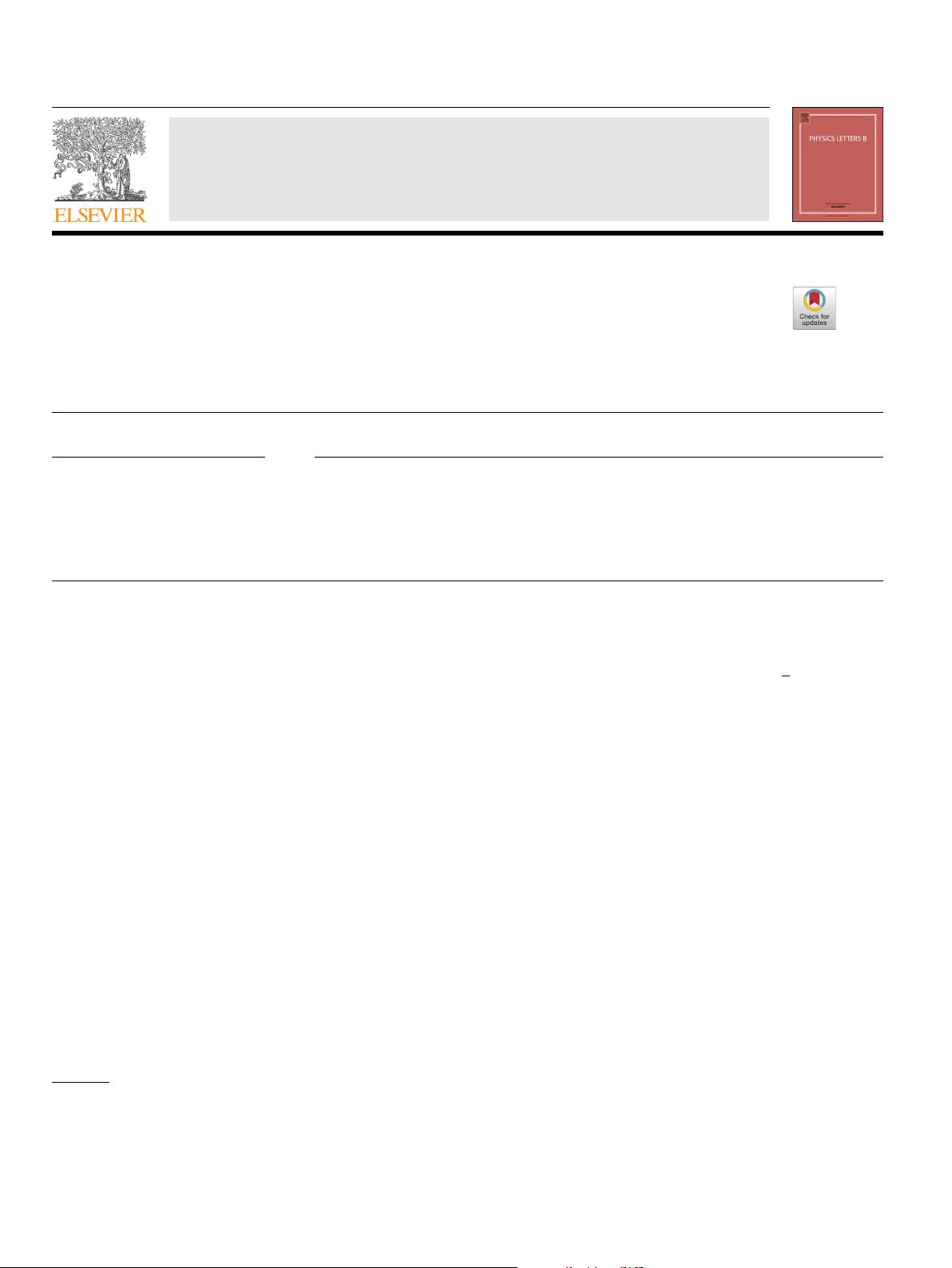
Physics Letters B 783 (2018) 158–162
Contents lists available at ScienceDirect
Physics Letters B
www.elsevier.com/locate/physletb
Gravitational radiation background from boson star binaries
Djuna Croon
a,∗
, Marcelo Gleiser
a
, Sonali Mohapatra
b
, Chen Sun
c,a
a
Department of Physics and Astronomy, Dartmouth College, Hanover, NH 03755, USA
b
Department of Physics and Astronomy, University of Sussex, Falmer, Brighton, BN1 9QH, UK
c
CAS Key Laboratory of Theoretical Physics, Institute of Theoretical Physics, Chinese Academy of Sciences, Beijing 100190, PR China
a r t i c l e i n f o a b s t r a c t
Article history:
Received
26 February 2018
Received
in revised form 19 March 2018
Accepted
19 March 2018
Available
online 22 March 2018
Editor:
M. Trodden
We calculate the gravitational radiation background generated from boson star binaries formed in locally
dense clusters with formation rate tracked by the regular star formation rate. We compute how the
frequency
window in gravitational waves is affected by the boson field mass and repulsive self-coupling,
anticipating constraints from EPTA and LISA. We also comment on the possible detectability of these
binaries.
© 2018 The Authors. Published by Elsevier B.V. This is an open access article under the CC BY license
(http://creativecommons.org/licenses/by/4.0/). Funded by SCOAP
3
.
1. Introduction
The recent detection of gravitational waves (GW) by LIGO and
VIRGO have opened up a new window for our understanding of
the physical properties of the universe [1]. Probing the energy den-
sity
of the stochastic Gravitational Wave Background (GRB) formed
by the superposition of a large number of individual gravitational
wave merger events is a long term goal of the next generation of
GW detectors. It is thus of great interest to investigate different
potential sources of GRBs and how to distinguish between their
potential observational signatures. In this letter, we compute the
GRB of an important class of hypothetical objects, merging bina-
ries
of Exotic Compact Objects (ECOs) composed of self-interacting
scalar field configurations known as boson stars (BSs). Such ob-
jects
were first proposed in the late 1960s [2] and further studied
in the 1980s and 1990s [3–6], but are now experiencing a revival
due to their potential role as dark matter candidates [7] and as
remnants of early universe physics [8]. The gravitational wave pro-
duction
from individual events of the merger of two boson stars
has been studied in [9] and [10], for example. A preliminary esti-
mate
of the GRB in boson-star binary mergers was given in [11].
The
success of inflationary cosmology [12] and the discovery
of the Higgs Boson [13][14]have opened up the possibility that
different self-interacting scalar fields might exist in nature. The
presence of such fundamental scalar fields in the early universe,
*
Corresponding author.
E-mail
addresses: djuna .lize .croon @dartmouth .edu (D. Croon),
mgleiser @dartmouth .edu (M. Gleiser), s .mohapatra @sussex .ac .uk (S. Mohapatra),
chen .sun @dartmouth .edu (C. Sun).
maybe in dark matter clusters, could have led to their condensa-
tion
into self-gravitating compact objects [15–17]. It is quite re-
markable
that for a repulsive self-interaction λ|φ|
4
and a scalar
field mass m, such objects have masses M
BS
∼
√
λM
3
Pl
/m
2
, which,
for m/λ
1/4
∼m
p
, where m
p
is the proton mass, are parametrically
equivalent to the Chandrasekhar mass [18].
Indeed, even a free, massive scalar field can generate a self-
gravitating
object, supported against gravitational collapse solely
by quantum uncertainty [2]. This distinguishes them from fermi-
onic
compact objects such as neutron stars (NS) and white dwarfs,
which are prevented from collapse due to degeneracy pres-
sure [19].
Another key difference, important observationally to
distinguish the two classes of compact objects, is that the sim-
plest
BSs do not radiate electromagnetically.
Given
the uncertainty in the details of BS formation, and to
provide a more general analysis, we assume here that BSs were
formed at a rate that tracks the regular star formation rate, in
locally-dense dark matter clusters. We will thus adopt this initial
range of redshifts as a benchmark for our analysis. Our results can
be extended to arbitrarily large redshifts.
As
with their fermionic counterparts, BSs have a critical maxi-
mum
mass against central density beyond which they are unstable
to gravitational collapse into black holes (BHs) [3,20]. In this pa-
per,
we treat the two stars in the binary BS system as having the
same maximum mass and radius, which leads to the two objects
having the same compactness, defined as C = G
N
M/R. The GRB
is typically characterized by the dimensionless quantity
GW
( f ),
the contribution in gravitational radiation in units of the criti-
cal
density in a frequency window f and f + δ f to the total
energy-density of the universe in a Hubble time. By studying their
gravitational imprints, we hope to gain insight on the properties
https://doi.org/10.1016/j.physletb.2018.03.055
0370-2693/
© 2018 The Authors. Published by Elsevier B.V. This is an open access article under the CC BY license (http://creativecommons.org/licenses/by/4.0/). Funded by
SCOAP
3
.