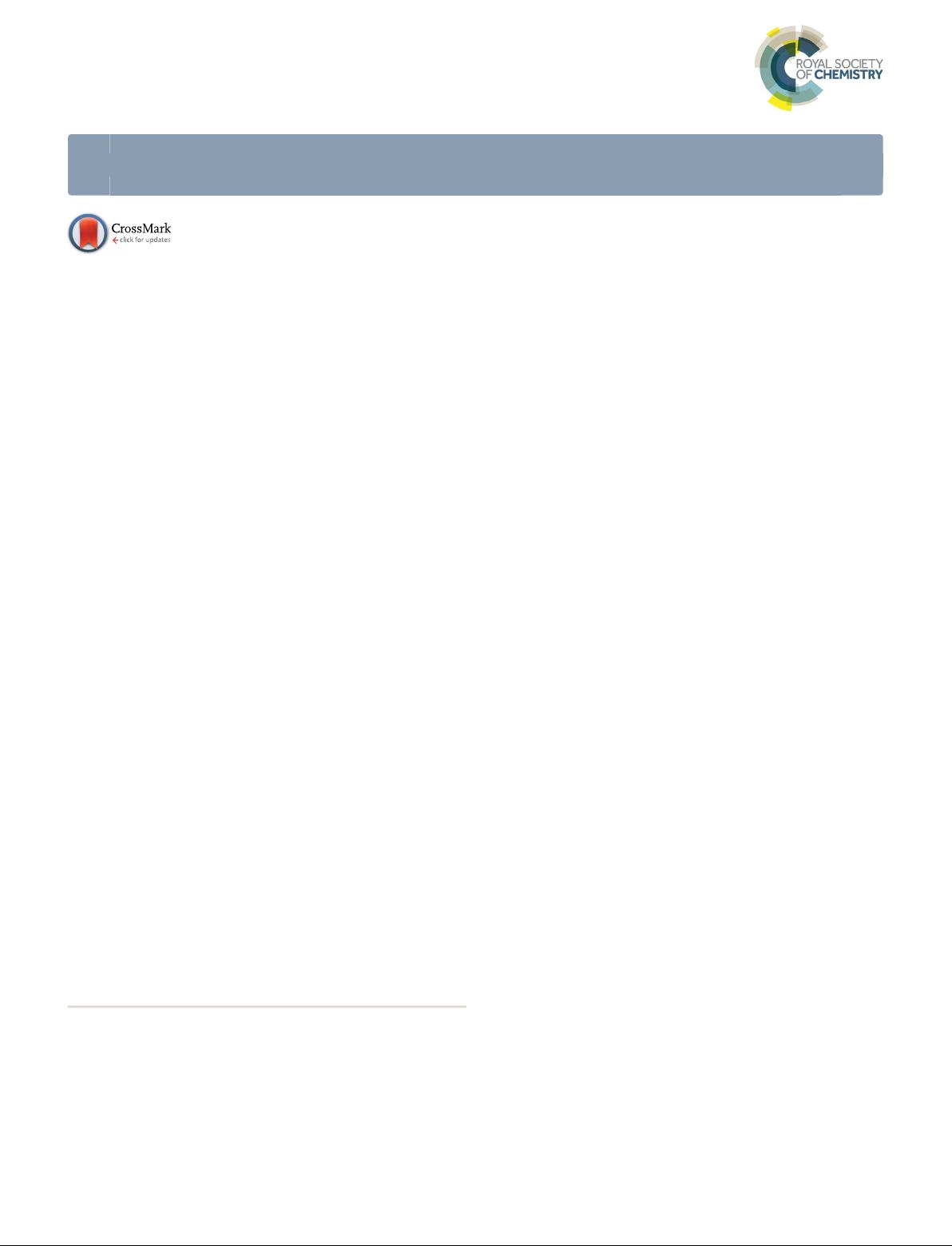
Hydrothermal growth and optical properties of
Nb
2
O
5
nanorod arrays†
Jing He,
a
Yongming Hu,
*
a
Zhao Wang,
a
Wei Lu,
b
Shulin Yang,
a
Guitai Wu,
a
Yu Wang,
b
Shengfu Wang,
a
Haoshuang Gu
*
a
and John Wang
c
Nb
2
O
5
nanorod arrays were grown on Nb foil through an in situ hydrothermal treatment process using
NH
4
F as the mineralizing agent and H
2
O
2
as the oxidant. The as-prepared Nb
2
O
5
nanorod arrays were
well crystallized with a hexagonal structure and a c-axis orientation. The effects of hydrothermal
temperature and concentration of NH
4
F on the growth of the nanorods were investigated. Nb
2
O
5
nanorod arrays are formed by crystal nucleation, oriented growth, followed by orientation attachment. A
higher concentration of NH
4
F accelerates the generation of Nb
2
O
5
nanorods as a result of corroding Nb
foil and releasing Nb ions and promotes the oriented growth of Nb
2
O
5
nanorods. The band gap of
Nb
2
O
5
nanorod arrays is measured to be about 3.3 eV with a blue light emission located at 456 nm
(2.719 eV) and a cyan light emission located at 490 nm (2.53 eV), respectively. The 2.53 eV peak can well
be attributed to the donor–acceptor pair (DAP) emission, and the 2.719 eV peak be related to the
conduction-band-to-acceptor transitions. There is only one quenching channel for 2.719 eV peak with
increasing temperature, which corresponds to the activation energy of about 16.9 meV, according to the
theoretical fitting.
Introduction
One-dimensional (1D) nanostructures of semiconductor oxides
promise great application potential in optical, electrical and
magnetic micro/nano-scaled devices such as eld emission
displays, energy conversion cells, high-density storage memo-
ries, microelectronic devices and chemical sensors.
1–4
Among
them, the wide band gap semiconductor oxides, such as ZnO
nanowires/nanobelts and TiO
2
nanorods/nanotubes, have been
extensively investigated owing to their excellent and particular
physical and chemical properties.
5,6
For instance, c-axis verti-
cally aligned ZnO nanorod arrays have been synthesized on ZnO
thin lm via hydrothermal route, which showed a high sensi-
tivity to H
2
at room temperature and a detection limit of 20
ppm.
7
However, ZnO is deliquescent and liable to degradation
due to moisture absorption, which would limit the practical
application of ZnO nanomaterials-based nanodevice under
harsh environments.
Niobium oxide is another important semiconductor oxide
with superior photocatalyst,
8
gas sensing,
9,10
electrochromic
11
and biosensing performance.
12
Among the different stoichi-
ometries of niobium oxide, Nb
2
O
5
is thermodynamically stable,
metal oxide of chemical inertness and low cytotoxicity. Up to
now, a variety of approaches have been employed for the growth
of Nb
2
O
5
1-D nanostructures, including thermal oxidation,
9
anodic oxidation,
13
plasma oxidation,
14
phase transformation
15
and a hydrothermal method.
16
Among them, the hydrothermal
method has been recognized as a simple, inexpensive and low-
temperature method for the growth of single-crystalline oxide
nanostructures. For example, single crystalline Nb
2
O
5
nanorods
were developed by a hydrothermal process at 200
C for
30 days using metal Nb powder and water as the starting
materials.
17
Based on this work, orthorhombic phased Nb
2
O
5
(O–Nb
2
O
5
) nanorod array lms with [001] orientation were
synthesized by the hydrothermal method in an ammonium
uoride (NH
4
F) aqueous solution environment, followed by
annealing treatment at 300–500
C.
16
However, the crystallinity
of the as-prepared hydrothermal samples is poor and needs to
be further improved by annealing at relatively high tempera-
tures; however, this can result in the secondary growth of the
grains and orientation changes. Moreover, the exact growth
mechanism and behavior of Nb
2
O
5
nanorods is unclear, which
may limit the controllable synthesis of nanorod arrays.
It has been reported that the addition of hydrogen peroxide
(H
2
O
2
) and NH
4
F simultaneously is important, which play a
pivotal role in the dissolution of metallic titanium, generating
a
Hubei Collaborative Innovation Center for Advanced Organic Chemical Materials,
Faculty of Physics & Electronic Science, Hubei University, Wuhan 430062, P.R.
China. E-mail: yongming.hu09@gm ail.com; guhsh583@hubu.edu.cn
b
Department of Applied Physics and Materials Research Centre, The Hong Kong
Polytechnic University, Kowloon, Hong Kong SAR, P.R. China
c
Department of Materials Science & Engineering, Faculty of Engineering, National
University of Singapore, BLK E3A, #04-10, 7 Engineering Drive 1, Singapore
† Electronic supplementary information (ESI) available. See DOI:
10.1039/c4tc01581a
Cite this: J. Mater. Chem. C,2014,2,
8185
Received 22nd July 2014
Accepted 5th August 2014
DOI: 10.1039/c4tc01581a
www.rsc.org/MaterialsC
This journal is © The Royal Society of Chemistry 2014 J. Mater. Chem. C,2014,2,8185–8190 | 8185
Journal of
Materials Chemistry C
PAPER