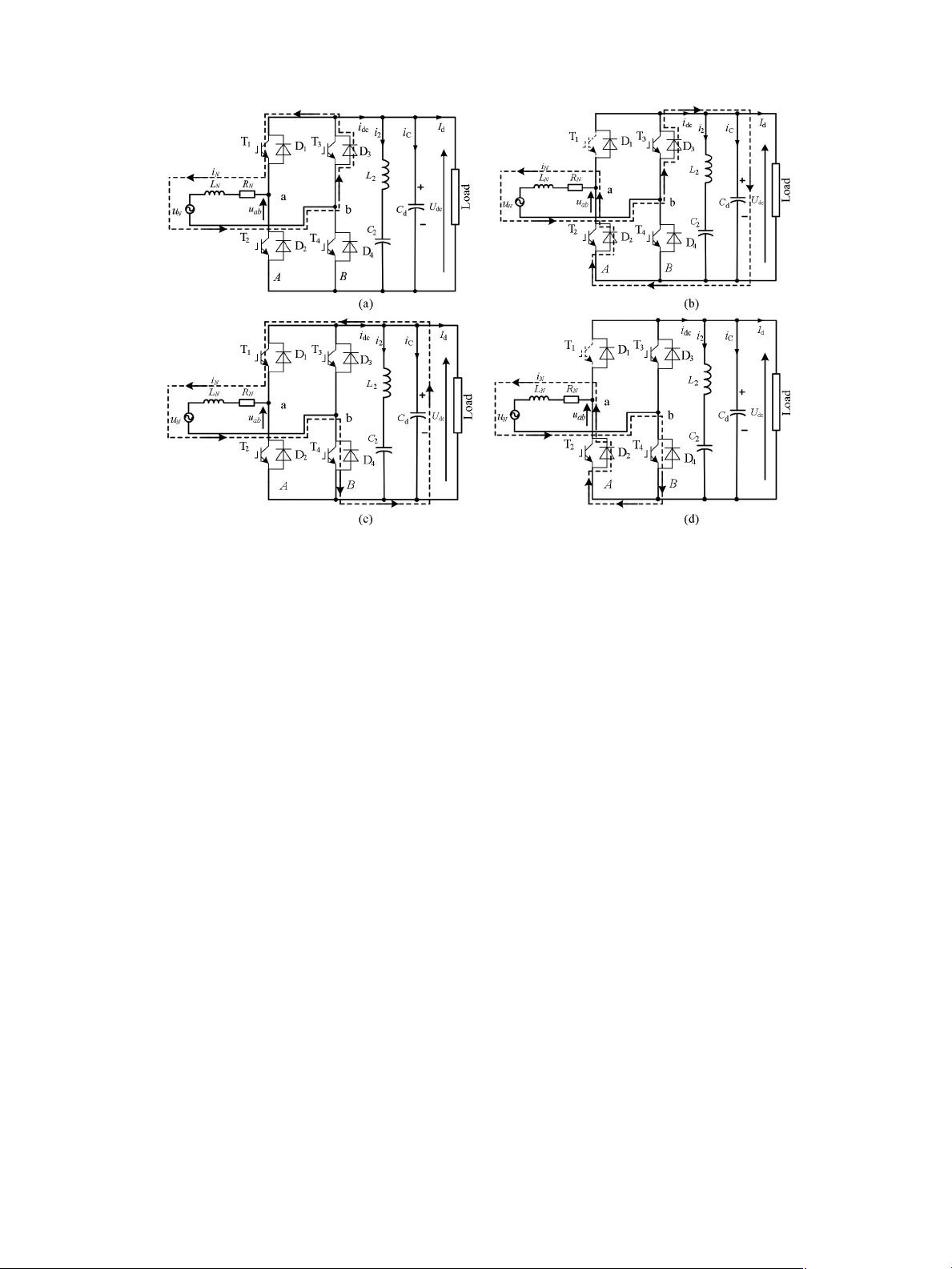
3818 IEEE TRANSACTIONS ON POWER ELECTRONICS, VOL. 31, NO. 5, MAY 2016
Fig. 3. Single-phase PWM rectifier circuit topology: (a) normal topology in command (1010) and (1000), (b) faulty topology in command (1010) and (1000),
(c) normal topology in command (1001), (d) faulty topology in command (1001), and the dash trace is the flowing direction of the catenary current.
open-circuit faults could have different fault characteristics and
result in different fault effects.
A. IGBT Open-Circuit Fault Analysis
In order to clearly analyze the IGBT open-switch fault con-
ditions in the single-phase PWM rectifier, it is assumed that the
upper switch of leg A is an open-circuit faulty switch, T
1
.Itis
assumed that the catenary current’s reference direction is from
the traction winding to the rectifier. As shown in Fig. 2, the
current could flow through the bypass diode D
1
associated with
the faulty switch when the catenary current is positive. Thus,
there is no change in the catenary current waveform during the
first half current cycle after the open-switch fault occurs. How-
ever, when the catenary current is negative, current cannot flow
through the bypass diode D
1
. If the catenary current is negative,
the rectifier cannot operate normally when the command signals
are (1010), (1001), and (1000).
Beginning with the command signal (1010), the upper switch
of leg A is turned on when the switch T
1
is in the normal
condition. The catenary current flows through the bypass diode,
D
3,
and switch, T
1
, shown as Fig. 3(a). In this context, the grid
voltage charges the inductor to facilitate energy storage, and the
amplitude of the catenary current is increasing. Meanwhile, the
energy stored in the dc-link capacitor is released to the load.
Thus, the dc-link voltage is reducing.
However, if switch T
1
in leg A is open circuited due to a fault
condition while its firing signal is high, the energy stored in the
inductor is released. Since the current flowing through an in-
ductor cannot change instantaneously, it must flow through the
bypass diode D
2
and D
3
, shown as Fig. 3(b). In this condition,
the grid voltage and the inductor charge the dc-link capacitor
simultaneously. Thus, the amplitude of the catenary current is
declining and the dc-link voltage is increasing. But, due to the
reducing of the catenary current amplitude, the increasing am-
plitude of dc-link voltage is limited, and depends on the load.
When the command is (1001), switch T
1
in leg A and switch
T
4
in leg B are turned on when T
1
not faulted, shown as Fig. 3(c).
In this case, both the grid voltage and the dc-link voltage charge
the inductor, resulting in energy storage. Therefore, the ampli-
tude of the catenary current increases sharply, and the dc-link
voltage is reducing.
But due to the open-circuit fault of switch T
1
, the catenary
current can only flow through the bypass diode D
2
and switch
T
4
, shown as Fig. 3(d). In this case, only the grid voltage charges
the inductor. Thus, the increasing amplitude of catenary current
is less than those in normal mode. Also, the energy stored in the
dc-link capacitor is released to the load. So, the dc-link voltage
is reducing.
With the command (1000), both the fault and the normal
mode are the same as command (1010). Since the signal (1000)
is effective only when the switch operates during the dead-time,
this command signal has little effect on the rectifier when there
is an open-circuit fault in T
1
.
Therefore, the amplitudes of the catenary current and the dc-
link voltage both are declining due to an open-circuit fault in T
1
,
shown as Fig. 4. All the previous explanations can be extended
to the remaining switches, T
2
,T
3
, and T
4
.
B. Diode Open-Circuit Fault Analysis
As for diode open-circuit fault, it is also assumed that the
upper diode of leg A is an open-circuit faulty diode, D
1
.As
shown in Fig. 2, the catenary current flows through the switch,