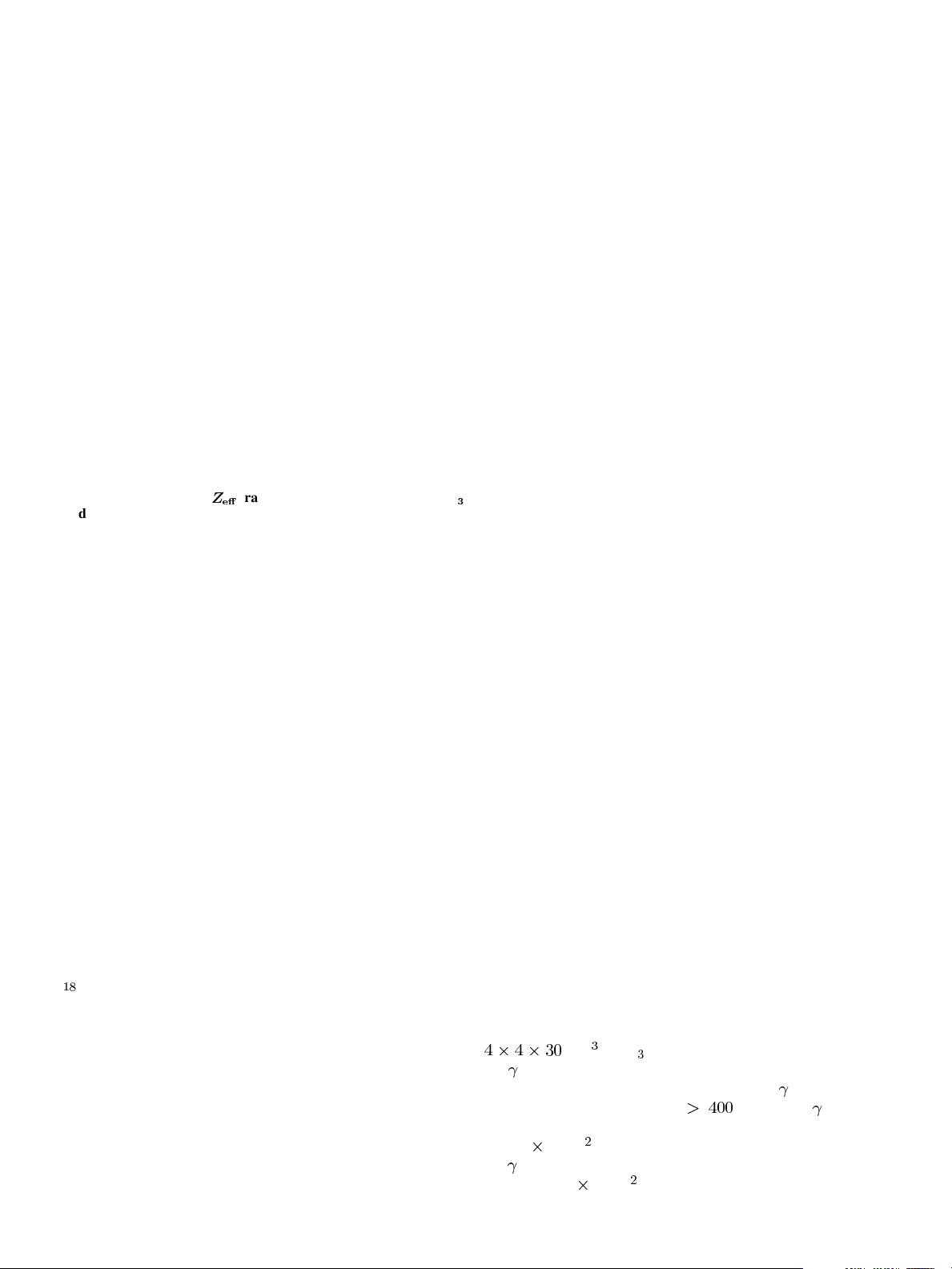
596 IEEE TRANSACTIONS ON NUCLEAR SCIENCE, VOL. 56, NO. 3, JUNE 2009
Improved Spatial Resolution in PET Scanners
Using Sampling Techniques
Suleman Surti, Senior Member, IEEE, Ryan Scheuermann, Matthew E. Werner, and
Joel S. Karp, Senior Member, IEEE
Abstract—Increased focus towards improved detector spatial
resolution in PET has led to the use of smaller crystals in some
form of light sharing detector design. In this work we evaluate two
sampling techniques that can be applied during calibrations for
pixelated detector designs in order to improve the reconstructed
spatial resolution. The inter-crystal positioning technique uti-
lizes sub-sampling in the crystal flood map to better sample the
Compton scatter events in the detector. The Compton scatter
rejection technique, on the other hand, rejects those events that
are located further from individual crystal centers in the flood
map. We performed Monte Carlo simulations followed by mea-
surements on two whole-body scanners for point source data.
The simulations and measurements were performed for scanners
using scintillators with
e
ranging from 46.9 to 63 for LaBr
3
and LYSO, respectively. Our results show that near the center
of the scanner, inter-crystal positioning technique leads to a gain
of about 0.5-mm in reconstructed spatial resolution (FWHM)
for both scanner designs. In a small animal LYSO scanner the
resolution improves from 1.9-mm to 1.6-mm with the inter-crystal
technique. The Compton scatter rejection technique shows higher
gains in spatial resolution but at the cost of reduction in scanner
sensitivity. The inter-crystal positioning technique represents a
modest acquisition software modification for an improvement
in spatial resolution, but at a cost of potentially longer data cor-
rection and reconstruction times. The Compton scatter rejection
technique, while also requiring a modest acquisition software
change with no increased data correction and reconstruction
times, will be useful in applications where the scanner sensitivity
is very high and larger improvements in spatial resolution are
desirable.
Index Terms—PET, pixelated detector, positioning, sampling,
spatial resolution.
I. INTRODUCTION
T
HE intrinsic physics limitations on reconstructed spatial
resolution in a PET scanner are determined by the positron
range which is a function of the positron energy [1], and the
non-collinearity of the two annihilation photons [2], [3]. For the
most common radio-isotope used in routine clinical imaging,
F, the positron range leads to a degradation of about 0.5-mm in
the system spatial resolution. Besides these two intrinsic physics
Manuscript received February 06, 2008; revised April 08, 2008. Current ver-
sion published June 10, 2009. This work was supported by the National Insti-
tutes of Health under Grants R33-EB001684 and R01-CA113941
S. Surti, M. E. Werner, and J. S. Karp are with the Department of
Radiology, University of Pennsylvania, Philadelphia, PA 19104 USA
(e-mail: surti@mail.med.upenn.edu; joelkarp@mail.med.upenn.edu;
matt.werner@uphs.upenn.edu).
R. Scheuermann is with the Department of Physics, University of Pennsyl-
vania, Philadelphia, PA 19104 USA (e-mail: rscheuermann@xrt.upenn.edu).
Digital Object Identifier 10.1109/TNS.2009.2013389
effects, a third factor in the system spatial resolution of a PET
scanner is the impact of the detector in localizing the inter-
actions of the annihilation photons within it. In recent years
there has been an increased focus in PET detector design to-
wards the use of small cross-section crystals in some form of a
light-sharing detector. Theoretically, assuming very high scin-
tillation photon statistics, the intrinsic resolution due to detector
effects is d/2 for a crystal of cross-section size, d. However,
the discrete (under) sampling due to fixed-size pixilated de-
tectors, leads to a convolution of the two effects [4]. The de-
tector sampling effect can be minimized with continuous scin-
tillation detectors that have very fine sampling within the de-
tector, and hence the detector resolution in this case is deter-
mined only by the uncertainty in positioning of the event within
the detector. This uncertainty is determined by the amount of
Compton scatter in the detector as well as the scintillation light
statistics. In order to improve the sampling in pixelated detec-
tors and achieve results closer to the theoretical estimates, re-
searchers in the past developed techniques such as “wobbling”
which involves a mechanical movement of the detector ring
while performing patient scans [5]–[7]. Our aim in this work is
to investigate two techniques that can be applied to the calibra-
tions performed in a pixelated detector design and potentially
lead to improved system spatial resolution in a PET scanner.
II. C
ALIBRATION TECHNIQUES FOR
POSITIONING EVENTS IN
ARRAYS OF SMALL
CRYSALS
In modern PET scanners based on pixelated detectors a
common technique is to use Anger logic positioning with en-
coding of the crystal to a PMT [8]–[10]. As a calibration step,
boundaries are drawn between all individual crystals in a flood
map and a look-up table generated which assigns all events
within a region (region-of-interest, ROI) to the center position
of that crystal [see Fig. 1(a) and (b)]. The center position is
the real physical position of that crystal on the scanner surface.
Fig. 1(b) shows schematically the crystal space partitioned into
ROIs where all points within each ROI are located closest to
that ROI peak.
We have performed simulations for a pixelated detector with
-mm LaBr crystals. For these simulations, only
the
-ray interactions were tracked and the interaction position
calculated as an energy-weighted mean of the
-ray interaction
points (total deposited energy
keV). The -ray entry
points were uniformly distributed over a single crystal surface
area (4
4-mm ). The energy weighted interaction position of
the
-rays was mapped onto a 2D array of ROIs, each of which
was initially 4
4-mm in area. The measured position of each
0018-9499/$25.00 © 2009 IEEE