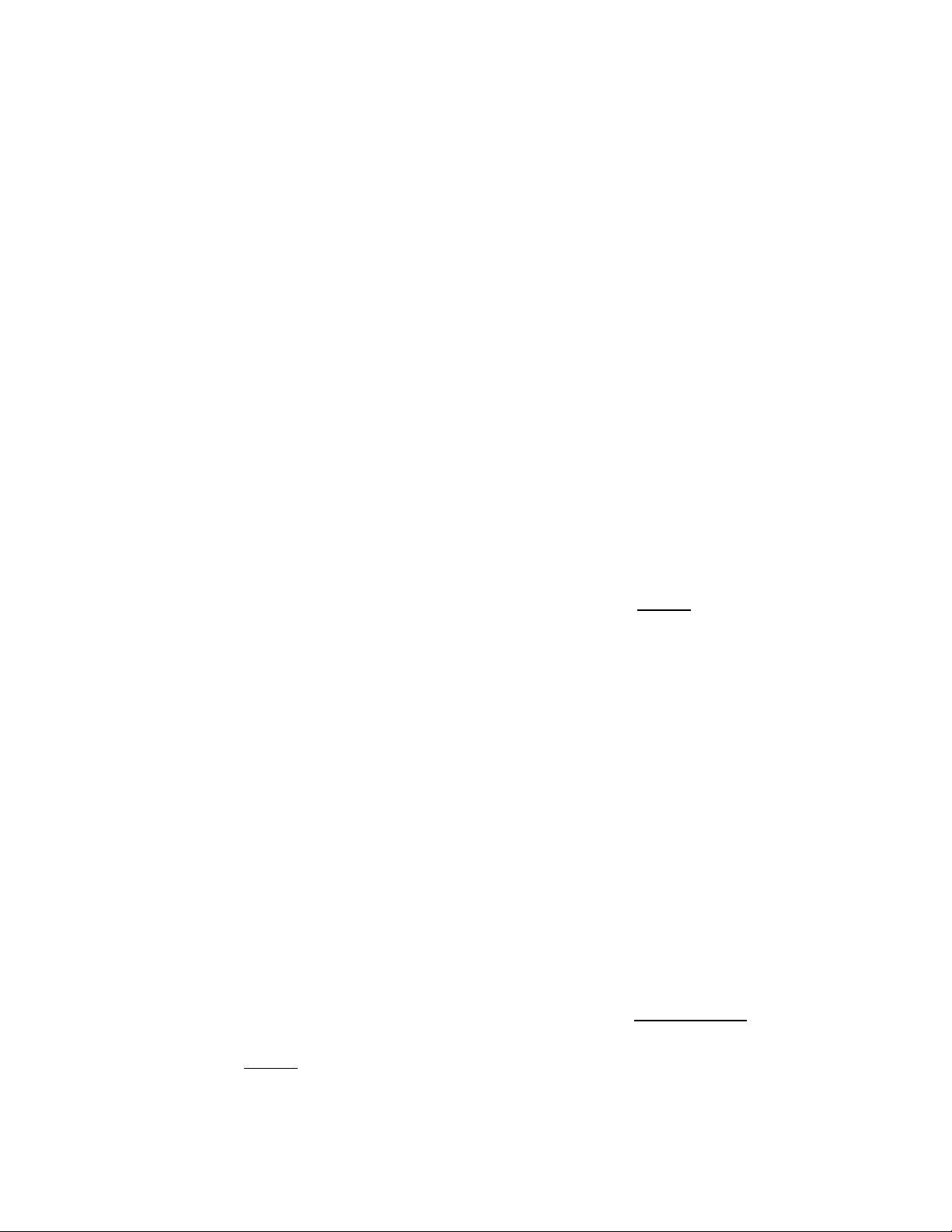
926 CHINESE OPTICS LETTERS / Vol. 8, No. 10 / October 10, 2010
Fluorescence lifetime imaging of molecular rotors to map
microviscosity in cells
(Invited Paper)
James A. Levitt
1
, Marina K. Kuimova
2
, Gokhan Yahioglu
2,3
, Pei-Hua Chung
1
,
Klaus Suhling
1∗
, and David Phillips
2
1
Department of Physics, King’s College London, Strand, London WC2R 2LS, UK
2
Department of Chemistry, Imperial College London, Exhibition Road, London SW7 2AZ, UK
3
PhotoBiotics Ltd., 21 Wilson Street, London EC2M 2TD, UK
∗
E-mail: klaus.suhling@kcl.ac.uk
Received June 13, 2010
Fluorescence liftime imaging (FLIM) of modified hydrophobic bodipy dyes that act as fluorescent molecular
rotors shows that the fluorescence lifetime of these probes is a function of the microviscosity of their
environment. Incubating cells with these dyes, we find a punctate and continuous distribution of the
dye in cells. The viscosity value obtained in what appears to be endocytotic vesicles in living cells is
around 100 times higher than that of water and of cellular cytoplasm.Time-resolved fluorescence anisotropy
measurements also yield rotational correlation times consistent with large microviscosity values. In this
way, we successfully develop a practical and versatile approach to map th e microviscosity in cells based on
imaging fluorescent molecular rotors.
OCIS code: 180.0180, 300.0300, 170.0170, 110.0110.
doi: 10.3788/COL20100810.0926.
Fluorescence imaging techniques are powerful tools in bi-
ological and biomedical sciences , because they are min-
imally invas ive and can be applied to living cells and
tissues. Fluoresc ence lifetime ima ging (FLIM) in partic-
ular has emerged as a key technique to image the envi-
ronment and interaction of specific pro teins and dyes in
living cells. It can report on photophysical events tha t
are difficult or impossible to observe by fluorescence in-
tensity imaging, because FLIM allows the separation of
fluorophore concentration and quenching effects
[1]
. The
vast majority of FLIM applications to date have been in
the biomedical and life sciences, as the technique is non-
destructive, minimally invasive and can be applied to liv-
ing cells and tissues
[2]
. The most frequent use of FLIM
is to detect F¨orster resonance energy transfer (FRET) to
identify pr otein interactions or conformational changes of
proteins
[3,4]
. Besides, applications in diverse are as such
as forensic science
[5]
, co mbustion research
[6]
, lumines-
cence mapping in diamond
[7]
, microfluidic systems
[8,9]
,
art conservation
[10]
, and lipid order problems in physical
chemistry
[11]
have also been reported. Moreover, efforts
are underway to use FLIM, possibly combined with en-
doscopy, for clinical diagnostics
[12]
. The power of FLIM
lies in the ability to remotely monitor the local environ-
ment of a molecular probe independent of the fluores-
cence intensity or local probe concentration
[1,13]
.
The fluorescence lifetime, τ
f
, is the average time a flu-
orophore remains in the excited state after excitation,
and is defined as the inverse of the sum of the rate pa-
rameters for all depopulation processes fro m the excited
state
[14,15]
:
τ
f
=
1
k
r
+ k
nr
, (1)
where k
r
is the radiative rate constant, and the non-
radiative rate constant k
nr
is the sum of the rate con-
stant for internal conversion (k
ic
) and the rate con-
stant for intersystem cross ing to the triplet state (k
isc
):
k
nr
= k
ic
+ k
isc
. The fluore scence lifetime is related to
the fluorescence quantum yield according to
Φ
f
=
k
r
k
r
+ k
nr
= k
r
τ
f
(2)
with 0< Φ
f
<1.
Diffusion is often an important rate-determining step
in chemical reactions or biological processes, and viscos-
ity is one of the key parameters affecting diffusion of
molecules and proteins. In biological specimens, changes
in viscosity have been linked to dis e ase and malfunction
at the cellular level, and signaling pa thways along with
protein-protein interactions are dependent on the trans-
port of biomolecules in cells. Elucidation of intracellular
reaction kinetics and mechanisms can thus potentially
assist in development and understanding of the mecha-
nisms of targeted therapies for cancer
[16]
.
While methods to measure the bulk viscosity are well
developed, macroscopic sa mple quantities are required,
and mechanical or fluid dynamics approaches are used
[17]
.
However, imaging the microviscosity, for example in sin-
gle cells, remains a challenge. Indeed, viscosity maps of
single cells have until recently been hard to obtain
[18,19]
.
Rotational diffusio n can be measured by time-resolved
fluorescence anisotropy r(t), which is defined as
[14,15]
r (t) =
I
||
(t) − GI
⊥
(t)
I
||
(t) + 2GI
⊥
(t)
, (3)
where I
||
(t) and I
⊥
(t) are the fluorescence intensity de-
cays parallel and perpendicular to the polarization of the
exciting light, and G acco unts for different transmission
and detection efficiencies of the imaging system at par-
allel and perpendicular polarization, and if necessary, an
1671-7694/2010/100926-05
c
2010 Chinese Optics Letters