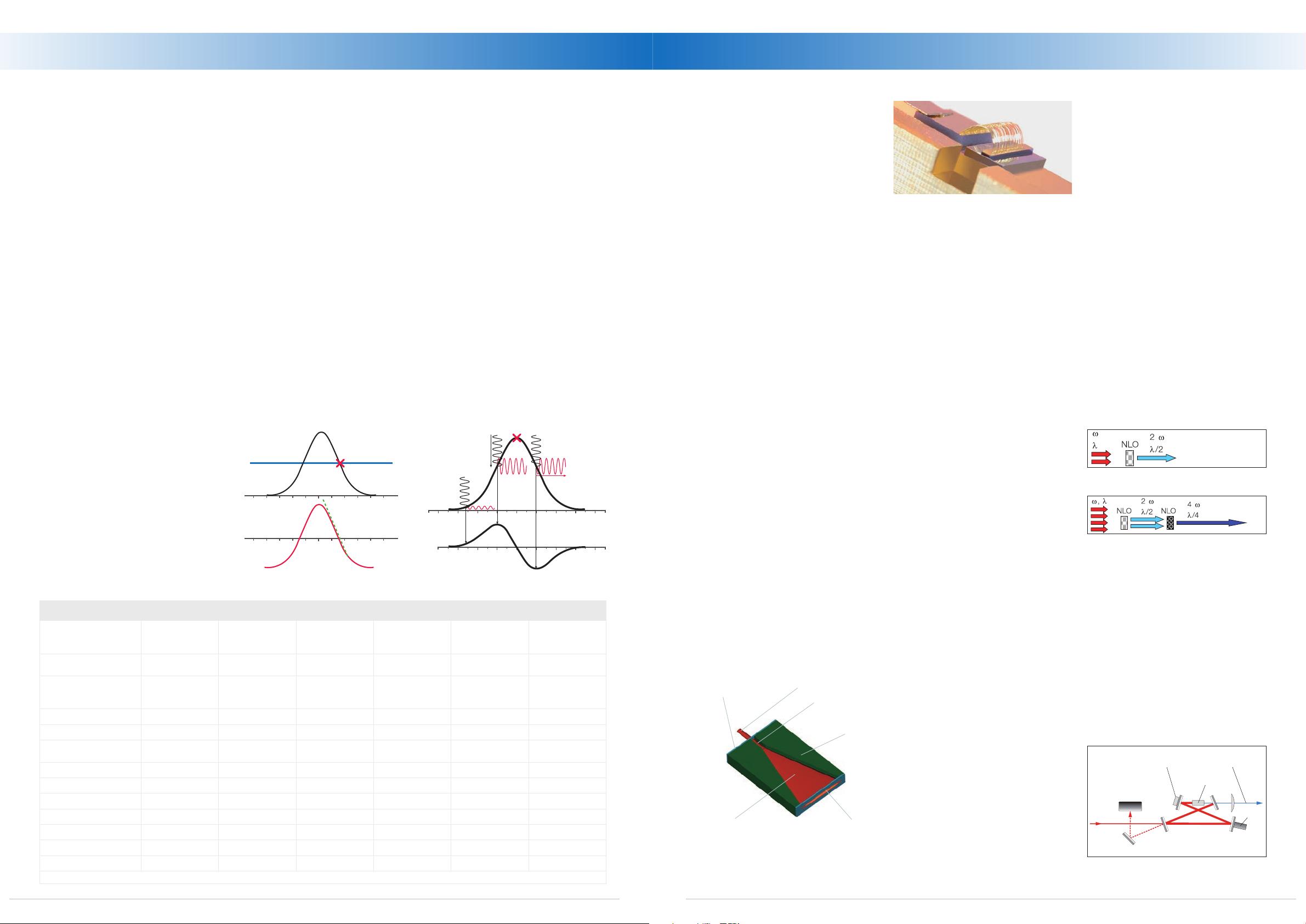
10
www.toptica.com www.toptica.com
11
DLC pro Lock* DigiLock 110 FALC 110 mFALC PID 110 PDD 110/F
Description / Type
PID regulator,
lock-in error
signal generator
PID regulator,
lock-in/PDH error
signal generator
PID regulator
mixing PID
regulator
PID regulator
PDH error signal
generator
Specials
all-digital, low
noise, convenient
versatile digital
locking solution
fast, analog,
for experts
fast, analog,
for experts
analog, slow
analog, high
bandwidth
Main application spectroscopy lock all
linewidth
narrowing
phase locking
side of fringe
spectroscopy
PDH locking, FM or
modulation transfer
spectroscopy
Side-of-fringe
Top-of-fringe
with PDD with PDD
Pound-Drever-Hall (PDH) slow with PDD
fast, super fast with
PDD
super fast with
PDD
with FALC, DigiLock,
DLC pro Lock, PID
Max. regulator bandwidth ≈ 30 kHz ≈ 10 MHz ≈ 45 MHz ≈ 45 MHz kHz .. 100 kHz ≈ 7 MHz
Modulation frequency 11 Hz .. 30 kHz 17 Hz .. 25 MHz 12 - 35 MHz**
Signal analysis FFT
Relock mechanisms
Computer control
High bandwidth output
No. of feedback channels 2 2 (+1) 2 2 1 n.a.
*software license for DLC pro **5- 70 MHz versions available
Frequency Stabilization
To actively frequency stabilize a laser, the
laser frequency is compared with a set
value, and a feedback circuit corrects any
deviations from this set value. Besides the
accuracy or the stability of the frequency
reference, an important parameter is the
rate of correction – in other words the
bandwidth of the control loop. It is limi-
ted by the time required for the frequency
comparison and the design of the feed-
back circuit, i. e. the used regulator modu-
les and the feedback elements. For active
linewidth narrowing or phase locking, one
even has to consider the lengths of the op-
tical beam path and the cables. Operation
convenience strongly favors digital locking.
Side-of-fringe lock
One can stabilize a laser to a spectrosco-
pic reference or the transmission of an FPI
with a “side-of-fringe” lock. Subtracting a
constant offset voltage from the original si-
gnal (“fringe”) such that the difference bet-
ween the two is around zero with non-zero
gradient an error signal is generated on the
side of the fringe. An appropriate locking
regulator adjusts feedback elements such
that the error signal in lock is zero and the
laser frequency is stabilized to the corres-
ponding side of the fringe zero-crossing of
the error signal.
Top-of-fringe lock
To lock to the maximum of a spectroscopic
signal (“top-of-fringe locking”), a signal
generator modulates the laser frequency
Principle of side-of-fringe (left) and top-of-fringe locking (right).
incident on a spectroscopy setup. The
observed signal is demodulated at the
modulation frequency with proper phase.
The resulting derivative of the spectros-
copic signal forms an error signal with
zero-crossing and non-zero gradient at the
frequency corresponding to the spectros-
copy maximum. Again, a locking regulator
stabilizes the laser frequency, this time to
the top of the fringe. The modulation can
be applied to the laser diode current or just
to the light of the reference setup using an
EOM or an AOM.
Linewidth narrowing & phase locking
One can reduce the linewidth of a laser and
even lock its optical phase to a reference
if the response of the locking circuit to de-
viations of the laser frequency/phase is
much faster than the speed at which these
changes occur. For linewidth narrowing,
one uses the Pound-Drever-Hall technique
together with high nesse resonators whi-
le optical phase locking is performed by
beating the laser with a second one or a
frequency comb. In both cases, the error
signal generation is extremely fast and one
can fully exploit the performance of special
high-bandwidth locking electronics.
TOPTICA locking modules
TOPTICA takes pride in having the lar-
gest portfolio of locking units with outper-
forming characteristics: highest speed,
maximum comfort, largest versatility, lo-
west noise and minimum drift. Whatever
is important and whatever locking task
has to be accomplished – the TOPTICA
solution is already there. A descripti-
on of our locking modules can be found
on pages 38-42 of this catalog and on
www.toptica.com. One can also use the
integrated PID option of our wavelength
meters if a laser has to be stabilized
to them with relatively low bandwidth
< 100 Hz, i.e. against drift.
Original signal
Offset
voltage
Difference
Linear
error signal
Derivative
Output (t)
Modulation (t)
Original Signal
Offset
voltage
Linear
error signal
Derivative
Output (t)
Modulation (t)
Original Signal
Amplifying laser diodes
Specially shaped semiconductor optical
ampliers, or Tapered Ampliers (TAs),
serve to increase the power available
from single-mode diode lasers in a
Master Oscillator Power Amplier (MOPA)
conguration. The master laser beam is
coupled into the single-mode channel
of the TA chip, which mainly acts as a
spatial mode lter. In the subsequent
tapered section, this “perfect” beam is
further amplied in single pass while freely
diverging in the tapered plane but being
conned in the orthogonal plane.
After exiting the large AR-coated output
facet of the amplier chip, this light can
be shaped again into a high-quality beam
with similar spectral quality as before.
Typically, a small-signal gain of up to 20 dB
and maximum output levels of up to 3.5 W
can be achieved.
TOPTICA is constantly working together
with renowned partners on TA chip
development. The main aims of this
initiatives are:
• to provide TAs chips at new wavelengths
• to improve the output power of TA chips
• to maintain the availability of TA chips
Examples for successful developments
are the extension of the NIR-A wavelength
limit from 1083 nm to 1357 nm by several
novel TA chips with multi-Watt output
power, the introduction of a new TA chip
for amplication around 690 nm and the
re-establishment of TA chips that reliably
provide > 500 mW at 670 nm. TOPTICA’s
high power diode lasers are presented on
pages 24-29.
Amplication & Frequency Conversion
Output facet,
AR coated
Tapered
amplier
Single-mode
channel
Injected beamEntrance facet,
AR coated
Tapered gain region
Geometry of a tapered amplier chip.
Frequency conversion
Despite the impressive success
story of semiconductor lasers, some
wavelength or power regions cannot
be directly accessed with current laser
diode technology. Nonlinear frequency
conversion techniques close these gaps.
TOPTICA’s frequency-converted laser
systems (pages 30-37) generate tunable
cw laser radiation in the DUV, UV, blue,
green, yellow, orange, and red spectral
ranges. We take pride in providing highest
power levels with unprecedented stability.
Second Harmonic Generation (SHG)
The SHG process can be explained in two
ways. In the wave picture, the fundamental
electromagnetic wave with frequency ω
1
drives the polarization of a non-linear optic
(NLO) crystal. Due to the non-linearity, the
polarization oscillates also at the “second-
harmonic” frequency ω
2
=2·ω
1
.
This causes the emission of a coherent
electromagnetic wave at the frequency
ω
2
. In the photon picture, two photons of
the fundamental laser wavelength λ
1
are
converted inside the NLO crystal into one
photon with half the original wavelength
λ
2
=0.5·λ
1
. The SHG efciency increases
with the fundamental power, the non-
linearity of the utilized crystal and proper
phase matching.
Phase matching: n(λ
1
) = n(λ
2
)
Phase matching implies that the refractive
indices n(λ) of the fundamental and the
frequency-converted light are equal within
the NLO crystal. This leads to a constructive
interference of second-harmonic partial
waves generated at different positions
within the crystal along the direction of
light propagation (wave picture). It also
guarantees momentum conservation:
Two photons of momentum p
1
= n(λ
1
)·h/λ
1
are converted into one photon of
momentum p
2
= n(λ
2
)·h/λ
2
=2·p
1
. Dispersion
usually prevents phase matching.
Amplier chip mounted on TOPTICA’s
proprietary heat sink.
Principle of Second Harmonic Generation (SHG).
Principle of Fourth Harmonic Generation (FHG).
Blue output
Piezo
NLO crystal
Intensity monitor
photodiode
Resonant doubling
cavity
Photodiode for
cavity stabilization
Second Harmonic Generator (sketch).
But it can be achieved using birefringent
crystals and different polarizations of
the light waves. Depending on crystal
type and operating wavelength, one can
ne-tune the phase matching by proper
adjustment of the crystal temperature or
the angle between crystal axis and light
propagation.
Resonant enhancement
TOPTICA’s SHG systems use a
compact rugged bow-tie resonator with
optimized mirror coatings to enhance the
fundamental laser power resonantly by a
factor of 50 to 400. For this, the resonator
length has to be actively stabilized with
respect to the fundamental wavelength.
Employing the Pound-Drever-Hall method
allows for tight locking with high long-
term stability and guarantees high power
with lowest noise gures. Together with
our best-of selection of NLO crystals, we
achieve highest conversion efciencies
and easiest handling in the market.
1
Scholz et al., Optics Express 20, 18659 (2012 )
2
Scholz et al., Appl. Phys. Lett. 103, 051114 (2013)
Fourth Harmonic Generation (FHG)
Fourth harmonic generation quadruples
the frequency of an electromagnetic
wave. TOPTICA’s FHG systems use two
consecutive SHG stages in one laser head.
In total, four photons of the fundamental
laser with frequency ω
1
are converted
into two photons with frequency ω
2
=2·ω
1
and then into one photon with frequency
ω
4
=2·ω
2
=4·ω
1
and wavelength λ
4
=0.25·λ
1
.
By this method, we have demonstrated
cw laser radiation down to 190 nm (1) with
more than 15 mW at 193 nm (2).