没有合适的资源?快使用搜索试试~ 我知道了~
首页2021年7月IEEE固态电路期刊:创新设计与应用论文精选
2021年7月IEEE固态电路期刊:创新设计与应用论文精选
需积分: 9 2 下载量 46 浏览量
更新于2024-07-07
收藏 131.75MB PDF 举报
《JSSC_202107》是IEEE固态电路期刊的一期,专注于每月发布的关于固体电路的广泛研究,特别强调集成电路的晶体管级设计。该期刊的内容涵盖了许多尖端的电路技术,这期特别关注以下几个重要主题:
1. 无晶振的GFSK调制BLE(蓝牙低功耗)数据包中的时钟恢复技术:论文由X. Chen、A. Alghaihab等人提出,他们设计了一种创新的系统,能在接收GFSK调制的BLE数据包时实现高精度的时钟恢复,这对于无线通信系统的高效工作至关重要。
2. 基于28纳米CMOS的145 GHz FMCW雷达系统及电路分析:A. Visweswaran等人分享了他们在毫米波雷达领域的研究成果,包括系统架构、电路设计以及性能验证,这对于无线传感和雷达应用具有重要意义。
3. 基带匹配电阻噪声取消接收器:A. N. Bhat等人介绍了一种仅采用三阶段运算放大器的接收器设计,旨在提高在宽频带和高增益输入信号下的信噪比(In-Band IIP3),适合于物联网应用中的信号处理。
4. 2.4 GHz高度选择性的IoT接收前端:论文讨论了如何通过优化功率管理的本地噪声温度放大器(LNTA)、频率分频器以及基带模拟滤波器来增强物联网设备的接收性能。
5. 直接RF发射器的时间近似滤波器:S. Su和M. S.-W. Chen设计了一种用于简化直接RF发射器设计的技术,可能涉及射频信号的精确时间处理和优化。
6. 四象限Class-G复数域多级数字功率放大器:S.-C. Hung、S.-W. Yoo和S.-M. Yoo共同研发的这一新型数字功率放大器技术,对提升无线通信系统的效率和性能有着显著贡献。
7. 低温环境下的宽带低噪声放大器:Y. Peng、A. Rufino和E. Charbon合作的论文展示了针对量子应用的低温条件下,如何实现低于1分贝噪声系数的宽带低噪声放大器,这是量子计算和通信领域的关键组件。
8. 结构工程学的前沿研究:最后,本期还包括了有关电路结构和设计的创新工作,这些可能涉及到新材料、新技术在固态电路中的集成与优化。
《JSSC_202107》提供了一个平台,让研究人员展示他们在高频、高速、低功耗和量子技术等领域的最新进展,这些成果将推动整个电子和通信行业的进步。
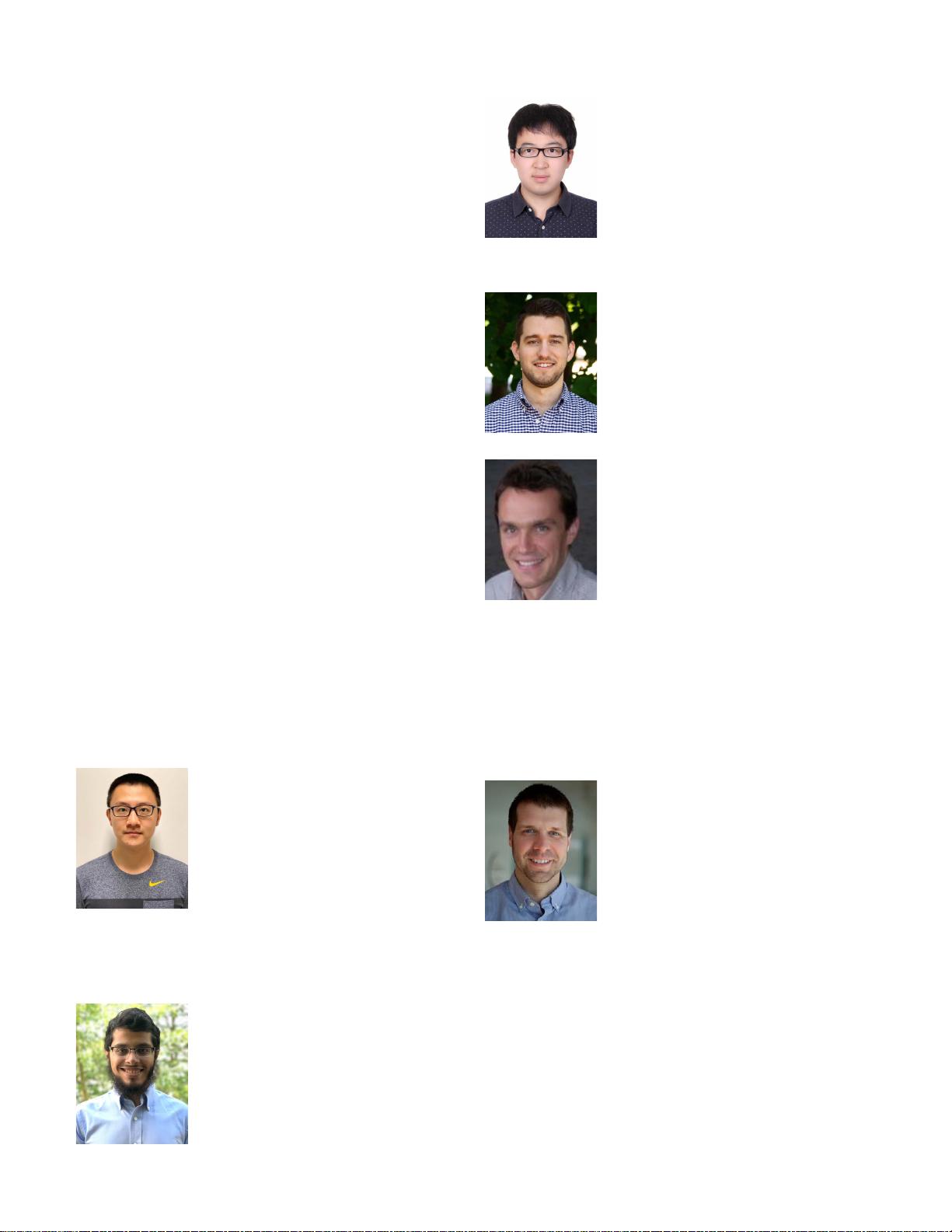
1974 IEEE JOURNAL OF SOLID-STATE CIRCUITS, VOL. 56, NO. 7, JULY 2021
[11] F. Maksimovic et al., “A crystal-free single-chip micro mote with
integrated 802.15.4 compatible transceiver, sub-mW BLE compatible
beacon transmitter, and cortex m0,” in Proc. Symp. VLSI Circuits,
Jun. 2019, pp. C88–C89.
[12] A. Alghaihab, X. Chen, Y. Shi, D. S. Truesdell, B. H. Calhoun, and
D. D. Wentzloff, “30.7 A crystal-less BLE transmitter with -86 dBm
freq μ ency-hopping back-channel WRX and over-the-air clock recovery
from a GFSK-modulated BLE packet,” in IEEE Int. Solid-State Circuits
Conf. (ISSCC) Dig. Tech. Papers, San Francisco, CA, USA, Feb. 2020,
pp. 472–474, doi: 10.1109/ISSCC19947.2020.9062935.
[13] A. Alghaihab, Y. Shi, J. Breiholz, H.-S. Kim, B. H. Calhoun, and
D. D. Wentzloff, “Enhanced interference rejection Bluetooth low-energy
back-channel receiver with LO frequency hopping,” IEEE J. Solid-State
Circuits, vol. 54, no. 7, pp. 2019–2027, Jul. 2019.
[14] X. Chen, “Analysis and design of energy efficient frequency synthesizers
for wireless integrated systems,” Ph.D. dissertation, Dept. Elect. Eng.
Comput. Sci., Univ. Michigan, Ann Arbor, MI, USA, 2019.
[15] A. A. Abidi, “Phase noise and jitter in CMOS ring oscillators,” IEEE
J. Solid-State Circuits, vol. 41, no. 8, pp. 1803–1816, Aug. 2006.
[16] X. Chen, H.-S. Kim, and D. D. Wentzloff, “An analysis of phase noise
requirements for ultra-low-power FSK radios,” in Proc. IEEE Radio
Freq. Integr. Circuits Symp. (RFIC), Honolulu, HI, USA, Jun. 2017,
pp. 37–40.
[17] X. Chen et al., “Analysis and design of an ultra-low-power Bluetooth
low-energy transmitter with ring oscillator-based ADPLL and 4× fre-
quency edge combiner,” IEEE J. Solid-State Circuits, vol. 54, no. 5,
pp. 1339–1350, May 2019.
[18] N. Da Dalt and A. Sheikholeslami, Understanding Jitter and Phase
Noise: A Circuits and Systems Perspective. Cambridge, U.K.: Cambridge
Univ. Press, 2018.
[19] W.-Z. Chen, T.-Y. Lu, W.-W. Ou, S.-T. Chou, and S.-Y. Yang,
“A 2.4 GHz reference-less receiver for 1 Mbps QPSK demodulation,”
IEEE Trans. Circuits Syst. I, Reg. Papers, vol. 59, no. 3, pp. 505–514,
Mar. 2012.
[20] Bluetooth, ‘Core System Package [Low Energy Controller],’ in Bluetooth
Core Specification V 5.0. Accessed: May 2020. [Online]. Available:
https://www.bluetooth.com/
[21] R. B. Staszewski et al., “All-digital PLL and transmitter for mobile
phones,” IEEE J. Solid-State Circuits, vol. 40, no. 12, pp. 2469–2482,
Dec. 2005, doi: 10.1109/JSSC.2005.857417.
[22] N. H. Sabah, Electric Circuits and Signals. Boca Raton, FL, USA:
CRC Press, 2017.
[23] E. Hegazi, H. Sjoland, and A. A. Abidi, “A filtering technique to lower
LC oscillator phase noise,” IEEE J. Solid-State Circuits, vol. 36, no. 12,
pp. 1921–1930, Dec. 2001, doi: 10.1109/4.972142.
Xing Chen (Member, IEEE) received the B.S.
degree from the Beijing Institute of Technology,
Beijing, China, in 2013, and the M.S. and Ph.D.
degrees from the University of Michigan, Ann
Arbor, MI, USA, in 2015 and 2019, respectively.
In 2019, he joined Qualcomm, San Diego, CA,
USA, as a Senior Engineer, where he is developing
frequency synthesizers and wireless transceivers for
5G applications. He also held internship positions at
Qualcomm, in 2018; Everactive, Inc., Ann Arbor,
in 2017; and Carnegie Mellon University, Pitts-
burgh, PA, USA, in 2014. His research interests include millimeter-wave/
RFIC design, frequency synthesizers, wireless transceivers, and energy-
efficient wireless integrated systems.
Abdullah Alghaihab (Member, IEEE) received the
B.S. degree in electrical engineering from King Saud
University, Riyadh, Saudi Arabia, in 2013, and the
M.S. and Ph.D. degrees from the University of
Michigan, Ann Arbor, MI, USA, in 2016 and 2020,
respectively.
He is currently an Assistant Professor with King
Saud University. His research interests include
RF/mixed-signal IC design for wireless communi-
cation and low-power wireless transceivers circuit
design and self-powered system on chip design for
the internet of things-based applications.
Yao Shi (Member, IEEE) received the B.S. degree
in electronic and information engineering from
Zhejiang University, Zhejiang, China, in 2013, and
the M.S. and Ph.D. degrees in electrical engineering
from the University of Michigan, Ann Arbor, MI,
USA, in 2016 and 2018, respectively.
From 2018 to 2020, he was a Design Engineer
with the MediaTek Inc., San Jose, CA, USA, devel-
oping radio frequency (RF) and millimeter-wave
(mm-wave) integrated circuit for WiFi and 5G wire-
less transceivers. Since 2020, he has been with
Apple Inc., Cupertino, CA, working on advanced display technology, system
architecture, and novel algorithm for future products.
Daniel S. Truesdell (Member, IEEE) received
the B.S. degree in electrical engineering from the
University of Central Florida, Orlando, FL, USA,
in 2016. He is currently pursuing the Ph.D. degree
in electrical engineering with the University of
Virginia, Charlottesville, VA, USA.
His current research interests include energy-
efficient mixed-signal circuit design, on-chip
oscillators and sensors, energy-harvesting and
performance-scalable systems, and low-power
digital circuit techniques.
Benton H. Calhoun (Senior Member, IEEE)
received the B.S. degree from the University of
Virginia, Charlottesville, VA, USA, in 2000, and
the M.S. and Ph.D. degrees in electrical engineer-
ing from the Massachusetts Institute of Technol-
ogy, Cambridge, MA, USA, in 2002 and 2006,
respectively.
He is currently a Professor with the Electrical
and Computer Engineering Department, University
of Virginia. He is also the Campus Director and
the Technical Thrust Leader with the NSF Nanosys-
tems Engineering Research Center for Advanced Self-Powered Systems of
Integrated Sensors and Technologies (ASSIST) Alexandria, VA. He has
coauthored the Sub-Threshold Design for Ultralow-Power Systems (Springer,
2006) and authored the Design Principles for Digital CMOS Integrated Circuit
Design (NTS Press, 2012). His research interests include body area sensor
nodes (BSNs), wireless sensor networks (WSNs), low-power digital circuit
design, subthreshold digital circuits, subthreshold field-programmable gate
arrays (FPGAs), static random access memory (SRAM) design for end-
of-the-roadmap silicon, power delivery circuits and architectures, variation
tolerant circuit design methodologies, and low-energy electronics for medical
applications.
David D. Wentzloff (Senior Member, IEEE)
received the B.S.E. degree in electrical engineer-
ing from the University of Michigan, Ann Arbor,
MI, USA, in 1999, and the S.M. and Ph.D.
degrees from the Massachusetts Institute of Tech-
nology, Cambridge, MA, USA, in 2002 and 2007,
respectively.
Since August 2007, he has been with the Univer-
sity of Michigan, where he is currently an Associate
Professor of electrical engineering and computer
science. In 2012, he co-founded PsiKick, a fab-
less semiconductor company developing ultralow-power wireless SoCs. His
research focuses on RF integrated circuits, with an emphasis on ultralow-
power design.
Dr. Wentzloff was a recipient of the 2009 DARPA Young Faculty
Award, the 2009–2010 Eta Kappa Nu Professor of the Year Award,
the 2011 DAC/ISSCC Student Design Contest Award, the 2012 IEEE Sub-
threshold Microelectronics Conference Best Paper Award, the 2012 NSF
CAREER Award, the 2014 ISSCC Outstanding Forum Presenter Award, the
2014–2015 Eta Kappa Nu ECE Professor of the Year Award, the 2014–2015
EECS Outstanding Achievement Award, and the 2015 Joel and Ruth Spira
Excellence in Teaching Award. He has served on the technical program
committee for ICUWB 2008–2010, ISLPED 2011–2015, S3S 2013–2014,
RFIC 2013–2020, and ISSCC 2020, and as a Guest Editor for the IEEE
T-MTT, the IEEE Communications Magazine,andtheElsevier Journal of
Signal Processing: Image Communication. He is a Senior Member of the IEEE
Circuits and Systems Society, the IEEE Microwave Theory and Techniques
Society, the IEEE Solid-State Circuits Society, and the Tau Beta Pi.
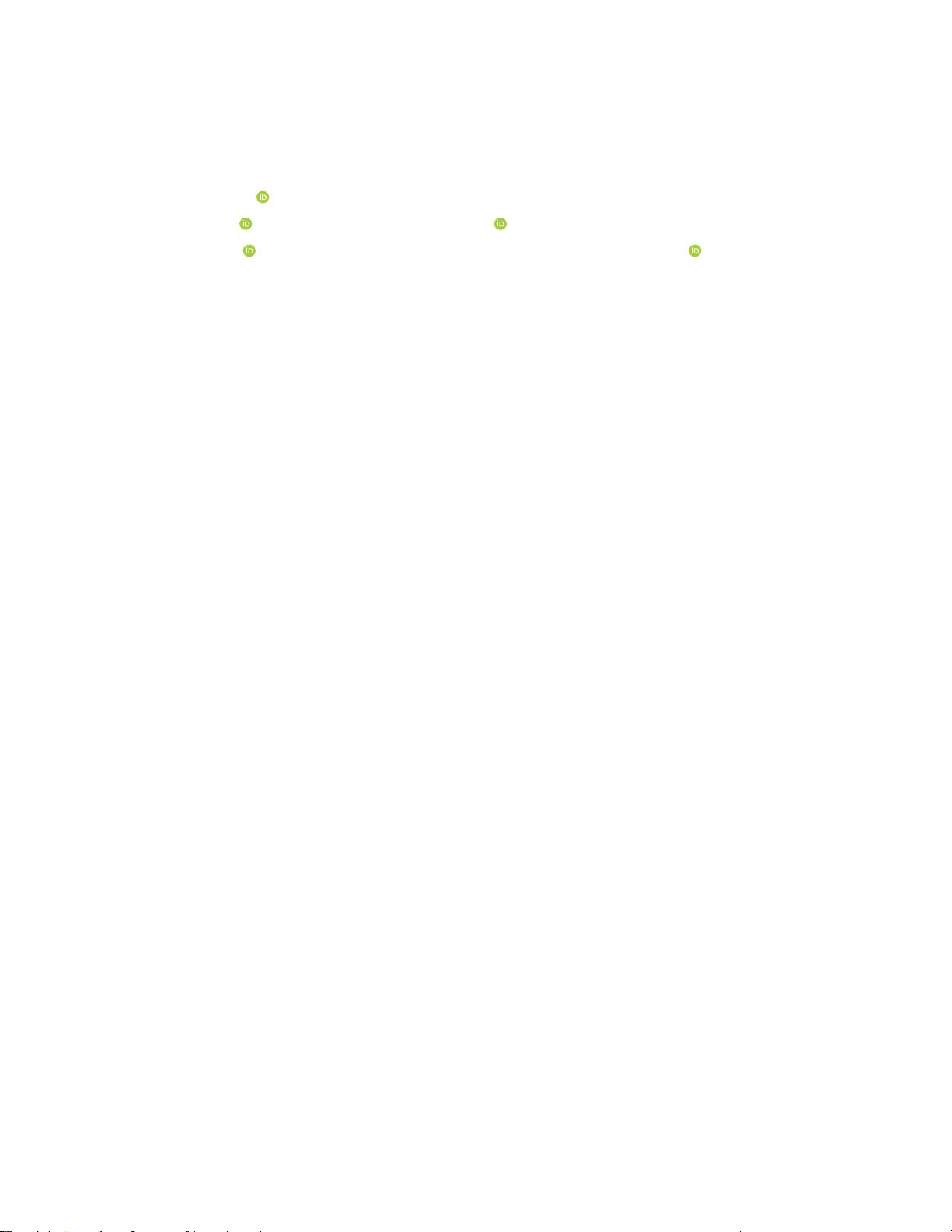
IEEE JOURNAL OF SOLID-STATE CIRCUITS, VOL. 56, NO. 7, JULY 2021 1975
A 28-nm-CMOS Based 145-GHz FMCW Radar:
System, Circuits, and Characterization
Akshay Visweswaran , Member, IEEE, Kristof Vaesen, Member, IEEE, Miguel Glassee, Member, IEEE,
Anirudh Kankuppe
, Member, IEEE, Siddhartha Sinha , Member, IEEE, Claude Desset, Member IEEE,
Thomas Gielen
, André Bourdoux, Senior Member, IEEE, and Piet Wambacq , Member, IEEE
Abstract—This article presents frequency-modulated-
continuous-wave (FMCW) radars developed for the detection
of vital signs and gestures using two generations of 145-GHz
transceivers (TRXs) integrated in 28-nm bulk CMOS. The
performance and limitations of high-frequency radars are
quantified with a system-level study, and the design and
performance of individual circuit blocks are presented in detail.
A 145-GHz center frequency and radar operation over an
RF bandwidth of 10 GHz yield a displacement responsivity
of 2π rad/mm and a windowed range resolution of 30 mm,
respectively. Radar operation over a 0.1–7 m range is enabled
by an effective-isotropic radiated power of 11.5 dBm and a noise
figure of 8 dB. The ICs feature frequency multiplication by 9 in
the transmit and receive paths, sub-arrayed dipole antennas,
and neutralization of TX–RX leakage via delay control. A single
TRX dissipates 500 mW from a 0.9-/1.8-V drive. The use of
fast chirps (5–30-μs) mitigates the effect of 1/ f -noise at the
intermediate frequency (IF). Extensive characterization results
showcase state-of-the-art performance of the TRXs, while
the code-domain multiple-input and multiple-output (MIMO)
radars (1×4and4× 4) built with them demonstrate vital-sign
and gesture detections.
Index Terms— D-band (110–170 GHz), frequency modulated
continuous wave (FMCW), gesture recognition, leakage neutral-
ization, link budget, on-chip antennas, radar.
I. INTRODUCTION
C
OMPACT high-resolution millimeter-wave radars,
resilient to fluctuations in ambient temperature and
light, are advancing the role of wireless systems in social
infrastructure. Radars in the 30–300-GHz range that have
catered historically to niche requirements in telemetry,
spectroscopy, and military reconnaissance [1] are finding
application in daily lives. These include automotive sensing,
imaging and security control in public spaces, and detection
of people and vital signs over short ranges [2]–[11]. Gesture
recognition for contactless man–machine interaction is an
exciting application of high-resolution radars ready to foray
into the mass market of smart, portable devices. Enabled
Manuscript received July 2, 2020; revised September 16, 2020 and
October 30, 2020; accepted November 24, 2020. Date of publication Janu-
ary 6, 2021; date of current version June 29, 2021. This article was approved
by Associate Editor Payam Heydari. This work was supported in part by
Panasonic, Japan, and in part by the European Commission’s Horizon 2020
under Grant 783119. (Corresponding author: Akshay Visweswaran.)
The authors are with imec, 3001 Leuven, Belgium (e-mail:
akshay.visweswaran@imec.be).
Color versions of one or more figures in this article are available at
https://doi.org/10.1109/JSSC.2020.3041153.
Digital Object Identifier 10.1109/JSSC.2020.3041153
by transistors that offer transit-frequencies beyond 350 GHz,
broad bandwidth and high fidelity outline the next milestones
of integrated radar technology.
Frequencies in the vicinity of 150 GHz favor broadband
radars as mm-scale resolutions can be obtained with fractional
bandwidths below 10%. The design is, therefore, amenable
to power-efficient RF techniques involving tuned circuits.
High-frequency carriers increase the angular resolution for a
given form factor and the displacement responsivity expressed
as phase rotation/displacement. They also facilitate compact
radars with antennas on the chip. From a system perspective,
correlation in frequency-modulated-continuous-wave (FMCW)
radars results in bandwidth compression at the intermediate
frequency (IF) [12]. This makes FM a low-complexity and
power-efficient approach for broadband-RF performance.
This article details radar systems for vital-sign and gesture
detections developed with two generations of 145-GHz
FMCW-radar transceivers (TRXs) integrated in 28-nm bulk
CMOS. The ICs include a single-input-single-output (SISO)
TRX with on-chip antennas [10] and its adaptation as a
dual-transmitter (dual-TX) single-receiver (single-RX) chipset
for tiling arrayed systems. A TRX bandwidth of 13 GHz
and a 145-GHz center frequency yield a minimum range
resolution of 11 mm and a displacement responsivity of
2π-rad/mm, respectively. These performance metrics increase
the discrimination capabilities for fine motions compared with
low-frequency radars while enabling smaller form factors. The
TRXs deliver 11.5 dBm of effective-isotropic radiated power
(EIRP), over a broad 3-dB bandwidth of 127–154 GHz. This
ensures the transmission of chirps with a constant amplitude
in the operating band. Integrated antennas and the LNA are
co-optimized for low-noise operation (8-dB noise figure), and
with 60 dB of programmable gain at IF, the TRXs also lend
themselves to short-range automotive sensing. The integrated
TRXs feature phase control for code-domain multiple-input
and multiple-output (MIMO) operation, TX-to-RX leakage
neutralization, the use of fast chirps (5–30 μs) to circumvent
1/ f -noise at IF, and a duty-cycled-FM transmission mode
for low-power operation. Complete MIMO-radar (1×4and
4×4) implementations using linear-FM chirps from the
sub-sampling PLL of [13] and an off-chip evaluation platform
are also showcased in this article.
Section II describes the performance and limitations of high-
frequency MIMO radars and the neutralization of TX–RX
leakage via delay control. The design of individual TRX
0018-9200 © 2021 IEEE. Personal use is permitted, but republication/redistribution requires IEEE permission.
See https://www.ieee.org/publications/rights/index.html for more information.
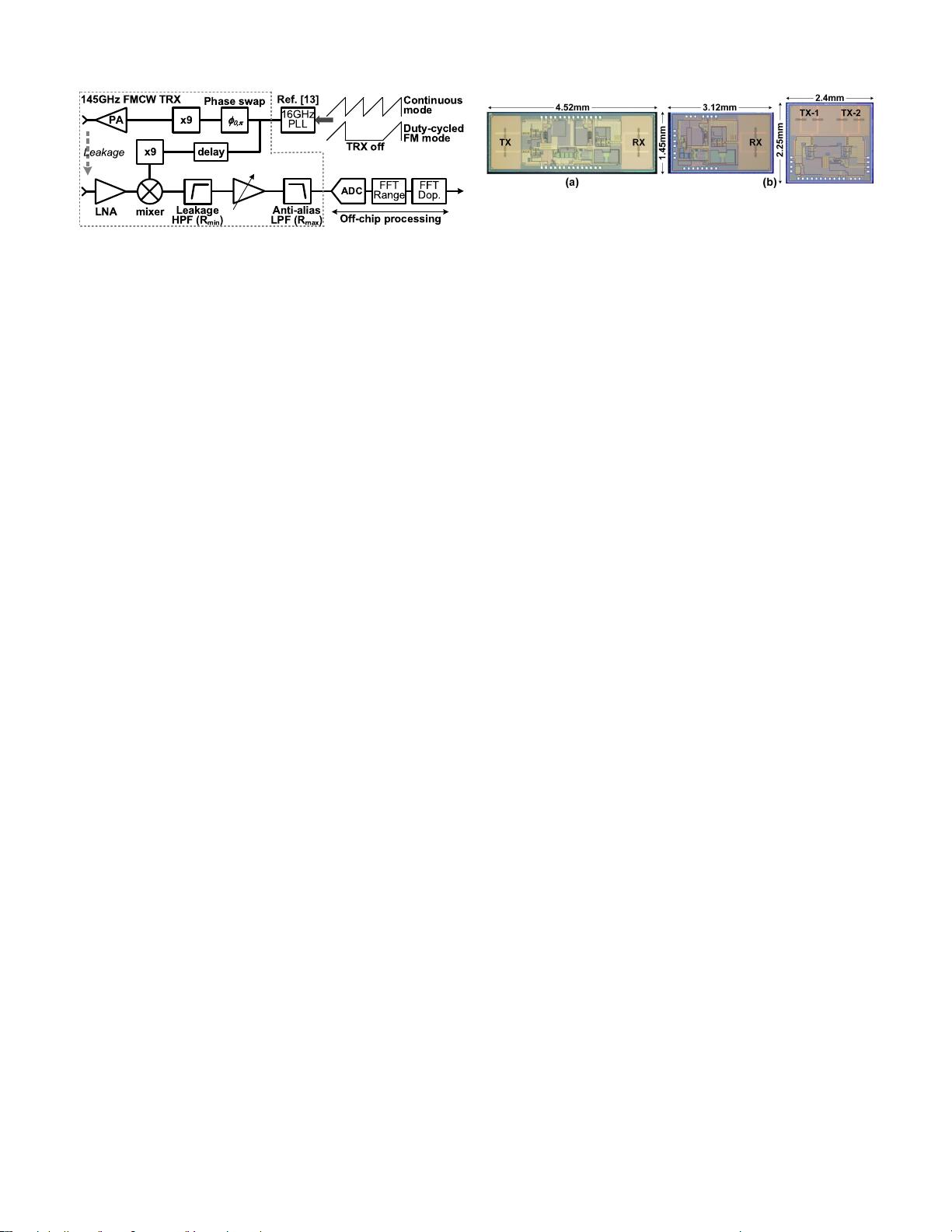
1976 IEEE JOURNAL OF SOLID-STATE CIRCUITS, VOL. 56, NO. 7, JULY 2021
Fig. 1. Simplified block diagram of the implemented radar system.
blocks and the on-chip antennas are detailed in Section III.
Section IV presents extensive characterization results of the
28-nm-CMOS TRXs and MIMO radars for the gesture and
vital-sign detection built using them. This work is summarized
in Section V.
II. FMCW R
ADAR SYSTEM
In linearly chirped FMCW radars, the correlation of
reflected and reference RF signals yields an IF tone pro-
portional to the time of flight over the target distance.
System-level analysis of broadband MIMO FMCW radars
and visual interpretation of the design space in this section
provide a comprehensive summary of the 145-GHz system
showcased here and the underlying tradeoffs and effects that
shape performance.
A. Overview of the Integrated Transceivers
A simplified block diagram of the 145-GHz radar TRX is
shown in Fig. 1. It has a 3-dB RF bandwidth of 138–151 GHz.
An external chirp signal centered at 16.1 GHz is upconverted
to 145 GHz via a cascade of two harmonic frequency triplers
in the RX and TX. In the radar context, broadband FM
components resulting from non-linearities demonstrate center
frequencies and modulation indices that are scaled by a
factor of the corresponding harmonic coefficient. Therefore,
while the bandwidth increases, the modulating waveform (i.e.,
the chirp function and its repetition interval) remains unal-
tered [14]. Despite operation at ∼ f
t
/2, thin metallization and
substrate losses affecting passives, and stringent density rules,
the TX EIRP is 11.5 dBm. The RX achieves a noise figure and
maximum conversion gain of 8 and 87 dB, respectively.
The radar operates over a 0.1–7-m range using fast chirps
of ∼5–30-μs duration (T
c
). The beat frequencies at IF fit
between the 400-kHz and 15-MHz bandpass corners designed
for 40-dB/decade high-pass and 120-dB/decade low-pass
roll-offs, respectively. Low-pass filtering suppresses noise
and aliasing above half the ADC sampling rate, f
s_ADC
,
of 80 MHz. The Nyquist-rate sampling yields a maximum
range of f
s_ADC
· c · T
c
/(4 · f
RF
) m. High-pass filtering, on
the other hand, attenuates the strong IF beat resulting from
TX–RX leakage. Apart from the time of flight in the leakage
path, group delays of the PA and LNA shift this beat into the
vicinity of the RX passband, which saturates the high-gain
RX. This is overcome by delaying the 16.1-GHz RX chirp
on-chip. Section II-E shows that delay compensation can
eliminate the leakage beat and its phase noise (PN) entirely.
Fig. 2. IC micrographs of (a) SISO TRX [10] and (b) the single-RX and
dual-TX chipset, integrated in 28-nm bulk CMOS.
Above 100 GHz, in-package antennas (using advanced
technologies, such as FO-WLP and eWLB) and integrated
antennas can enable efficient, broadband radiation of power
generated on the chip. The optimal choice is driven by an
application-based survey of performance, cost, complexity, and
yield. This work explores sub-arrayed dipoles on the chip
that mitigates deleterious substrate modes and radiates at 62%
efficiency. The radar fields of view are 100
◦
and 80
◦
in the
E-andH-planes, respectively.
Micrographs of the SISO TRX and its adaptation as a dual-
TX, single-RX chipset for tiling arrayed radars are shown
in Fig. 2(a) and (b), respectively. The RX in both versions is
identical. In the SISO TRX, spatial power combining results
from individual PA drive of the antenna sub-elements. For
layout compactness in the dual-TX chip, a single PA drives
the dipole sub-elements. The TRXs and on-chip antennas are
presented in Section III along with detailed block diagrams.
MIMO configurations typically entail antenna spacings of
multiples of λ
0
/2 (i.e., 1.1 mm at 145 GHz), depending on the
number of TX/RX elements. An additional physical separation
is often kept between TX- and RX-element clusters to mini-
mize crosstalk. Therefore, with an integrated-antenna solution,
a multi-chip approach utilizing less silicon area than a fully
integrated MIMO radar proves to be cost-efficient. Coherent
detection subsequently necessitates a central chirp distribution
network that stems from an FM source external to individual
TX/RX elements in the array. To circumvent the distribution of
mm-wave signals, a linear chirp centered at 16.1 GHz from the
sub-sampling PLL developed for this work [13] is distributed
to multiple TRX chips on a PCB. MIMO radars implemented
with the ICs of Fig. 2 are detailed in Section II-D.
B. Link Analysis: Overcoming Negative SNR
The link budget for the gesture and heartbeat detection
is presented in this section. We would like to notify the
reader at the outset that numerous variables quantify the
system parameters of Table I. Although they are introduced
in the text when first referred to, all variables and their units
are listed alphabetically in Table II for easy referencing at
any point.
The transmit power, P
TX
, at 145 GHz is limited by the
technology, and the IF bandwidth is determined by the ADC
sampling rate. Table I considers a maximum transmit power
of 10 dBm per TX, antenna gains of 2 dBi, and an 80-MHz
sampling rate. The RX noise figure is 8 dB, while 10 GHz of
RF bandwidth yields a windowed range resolution of 27 mm
[see (3)]. The 145-GHz carrier frequency yields a displacement
responsivity of 2π rad/mm [see (4)]. The upper cutoff at IF
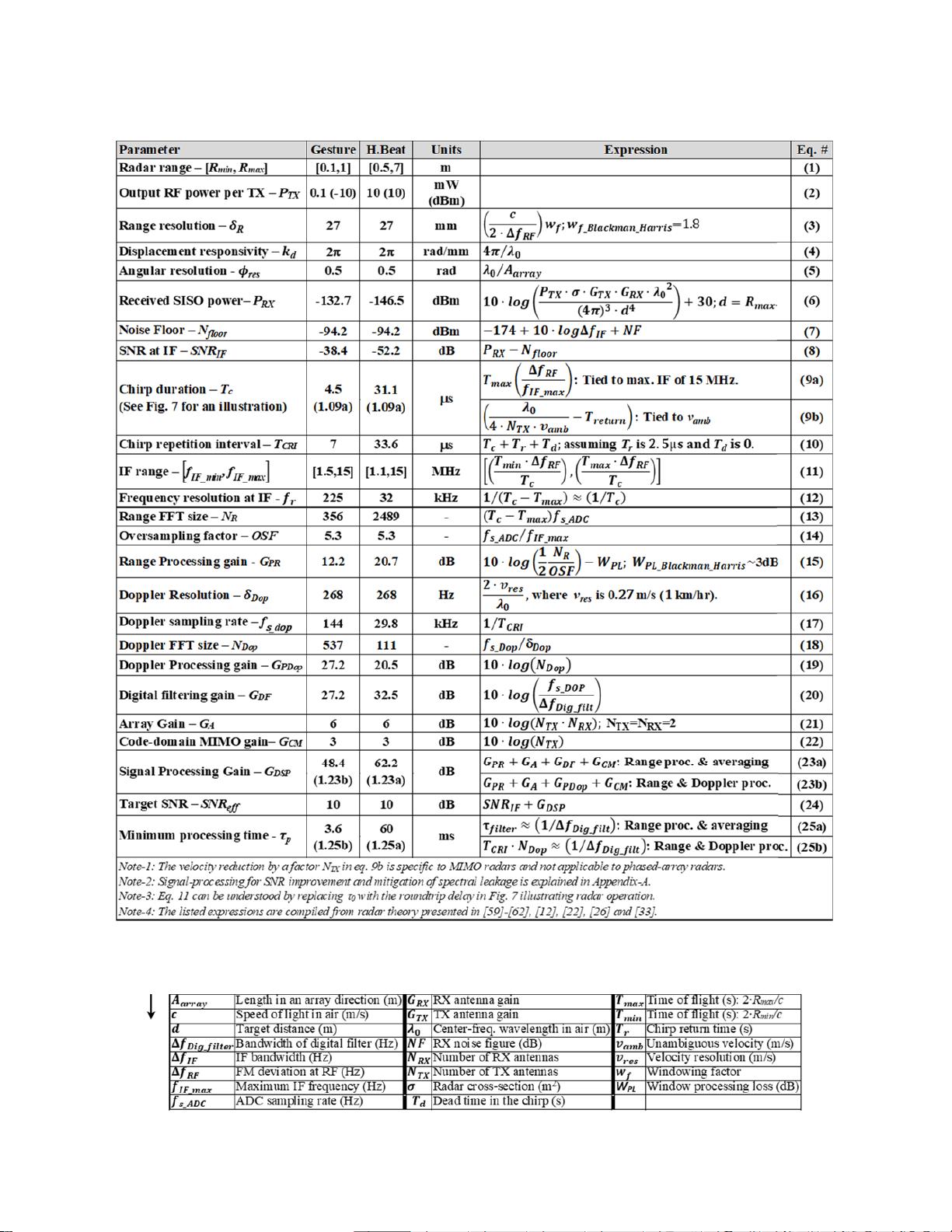
VISWESWARAN et al.: 28-nm-CMOS BASED 145-GHz FMCW RADAR: SYSTEM, CIRCUITS, AND CHARACTERIZATION 1977
TABLE I
L
INK BUDGET OF AN FMCW RADAR FOR GESTURE AND HEARTBEAT DETECTION (−40-dBsm RCS; N
tx
, N
rx
= 2; AND f
RF
= 10 GHZ)
TABLE II
L
IST OF VARIABLES IN TABLE I AND THEIR UNITS IN ALPHABETICAL ORDER (PHONETICS IN THE CASE OF GREEK LETTERS)
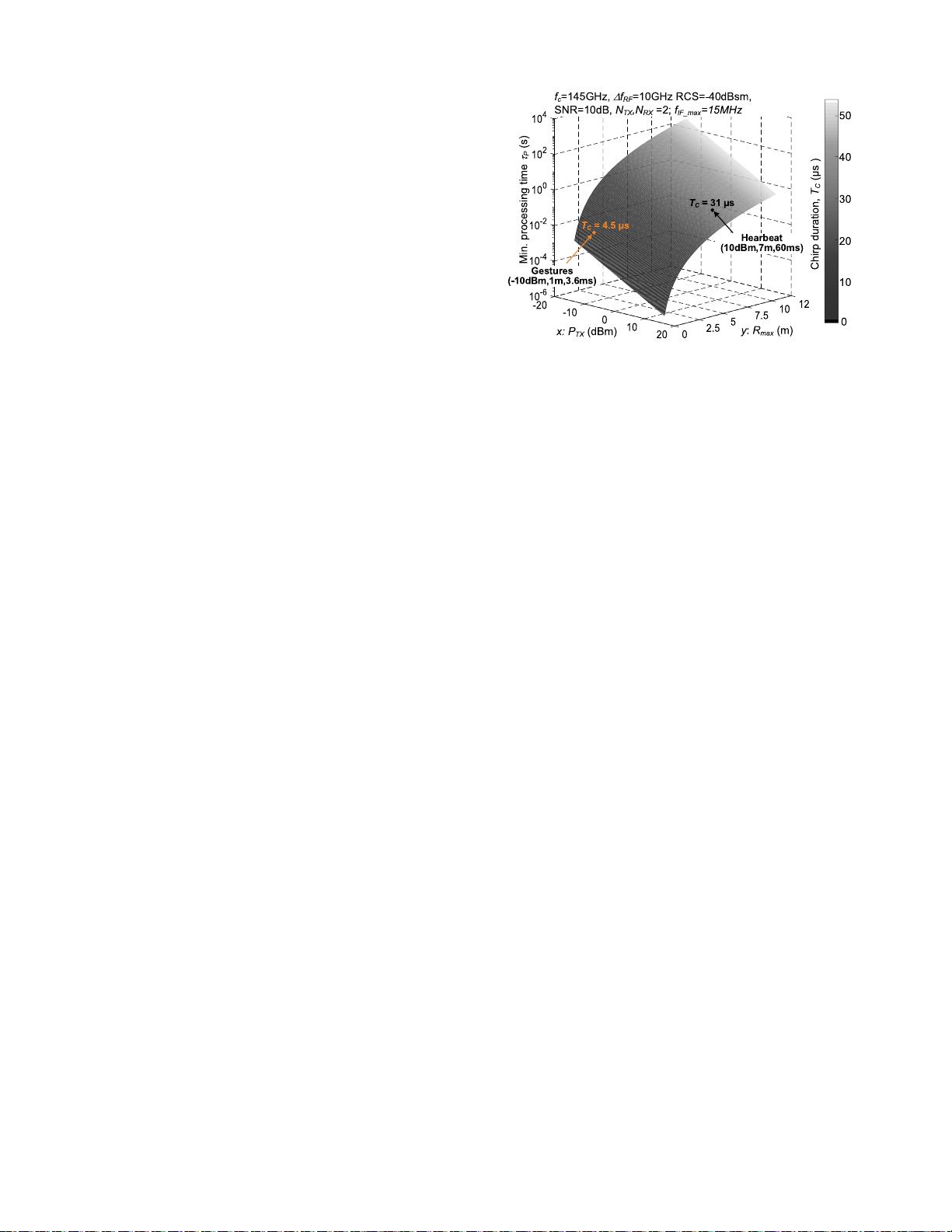
1978 IEEE JOURNAL OF SOLID-STATE CIRCUITS, VOL. 56, NO. 7, JULY 2021
is 15 MHz [see (11)], leaving a margin for filtering aliases
and noise above f
s_ADC
/2 [see (14): OSF > 5)]. More than
45-dB suppression is achieved at 40 MHz via the sixth-order
low-pass filtering on-chip. The chirp duration is calculated
for objects at maximum range, R
max
[see (1)], to map to a
maximum IF, f
IF_max
, of 15 MHz via [see (9a)]. The requisite
fast chirps of 5–30-μs duration circumvent SNR degradation
due to flicker noise due to the relatively high IF range [see
(11)]. The sawtooth modulation comprises the chirp duration,
T
c
, and the return time, T
r
[see (10)]. Additional dead time,
T
d
, may be included between chirps to aid PLL settling; it
is assumed to be zero here. Fast modulation also increases
the maximum unambiguous velocity, v
amb
[see (9b)], and the
separation at IF is seen between targets of varying radar-cross
sections (RCSs) in proximity.
The link analysis reveals a significant loss in power
attributed to pathloss and an RCS of −40 dBsm [see (6)].
Movement of the thoracic chest induced by heart-
beats [15]–[17] is characterized in-house using a commercial,
79-GHz radar [18]. The RCS varies from −37 to −40 dBsm.
The RCS of a hand characterized at 60–90 GHz ranges
from −20 to −45 dBsm [19]. These match our estimates
of −38 to −42 dBsm. The representative value of −40 dBsm
in Table I is nearly five and four orders of magnitude lower
than automobiles and people, respectively [20], [21], which
is something that highlights the detection challenge.
Despite the compression of the noise bandwidth at IF
[see (7)], both scenarios encounter negative SNRs [see (8)].
Array and signal-processing gains [see (13) and (19)–(22)]
improve SNR after the ADC and are an integral part of
detection [see (23)]. They are explained in Appendix A. The
range-processing gain simplifies to R
max
/(2 · δ
R
) when (9a)
is satisfied, and it is assumed that f
RF
-f
IF_max
≈ f
RF
.
The finer the range resolution (δ
R
), the better the SNR
per range bin is. In this work, the Blackman–Harris win-
dowing (sidelobe suppression >60 dB) is applied to the
range-processing FFT step, which enables target discrimina-
tion amid the clutter. A windowed DFT degrades the range res-
olution and SNR by a factor determined by the filter type [22].
Here, a windowing factor of 1.8 increases the resolution to
27 mm [see (3)] and introduces a ∼3-dB SNR degradation
[see (15)].
When the chirp duration is viewed in terms of [see
(1.09b)], the Doppler processing gain [see (19)] simplifies
to (v
amb
/v
res
), assuming T
c
+ T
r
≈ T
c
.However,inthe
heartbeat scenario in Table I, we employ digital filtering (refer
to Appendix A for details) after the first FFT, which dominates
the minimum processing time [see (25a)] required to meet a
viable-SNR threshold of 10 dB [see (24)]. Micromotions, such
as heartbeats and respiration of a static person, which do not
shift the range bin, result in a phase variation at IF determined
by the displacement responsivity [see (3)]. A motion of half
a wavelength (i.e., 1 mm) corresponds to a two-way phase
rotation of 360
◦
. The motion is typically detected as a change
in phase at IF in the range bin corresponding to the target
location, using successive Doppler FFTs and observing the
change in the argument of the complex FFT value in the
first/dc Doppler bin. Gesture recognition using R-D maps
Fig. 3. Application space of the 145-GHz radar, parameterized by TX power,
range, minimum processing time, and chirp duration, for 15 MHz of f
IF
,
10 GHz of f
RF
, and a target SNR of 10 dB.
and machine learning is explained in Section II-G. Radar
measurements are shown in Section IV.
C. Application Space and Limitations at 145 GHz
The detection of gestures and vital signs with 10-dB SNR
at distances of 1 m and 7 m, respectively, lies on diametric
ends of the design space parameterized by transmit-power
per TX, radar range, and minimum processing time (see
Fig. 3). The heartbeat detection with 10-dBm P
TX
entails a
minimum processing time of 60 ms, while the gestures with
-10 dBm of P
TX
require 3.6 ms. For radar to efficiently fit
multiple scenarios, it requires: 1) flexibility in T
c
to operate
over a constant IF bandwidth
1
[grey scale in Fig. 3, see
(11)] and 2) flexibility in the output power. The first require-
ment is met with the PLL of [13]. The latter is, however,
impeded by the diminishing back-off efficiency of power
amplifiers (PAs) at 145 GHz. State-of-the-art SiGe-BiCMOS
and FinFET-CMOS PAs achieve power-added efficiencies of
∼13% at saturated output power (P
sat
) levels of 15 and
18 dBm, respectively [23]–[25]. The 28-nm CMOS PA devel-
oped here delivers 7 dBm at 7% PAE. The desired radar
operation at peak efficiency, even at low output power levels,
is enabled by duty-cycled FM transmission (see Fig. 1), where
the TRX is switched
OFF between the chirps or a set of
chirps. It should be noted that, irrespective of waveform type,
the actual observation time determines the Doppler resolution.
While short- and mid-range applications at high frequen-
cies benefit from improved fidelity, broadband operation, and
small form factors, the detection of larger objects, people,
and vehicles over longer ranges encounters limitations in
SNR and velocity tracking. For a fixed field of view (i.e.,
antenna directivity) and P
TX
(10 dBm here), the range of
operation increases with the array gain. Fig. 4(a) shows that
a four-element virtual array can detect larger targets (e.g.,
persons with an RCS of 5 dBsm) over nearly 47 m with
10-dB SNR. Larger ranges require a higher IF bandwidth,
1
Depending on complexity and needs, a flexible IF band or a combination
of programmable chirp duration and IF band may also be used.
剩余335页未读,继续阅读
2014-09-15 上传
2012-12-21 上传
2021-12-26 上传
2021-12-26 上传
2021-12-26 上传
2021-12-26 上传
2021-12-26 上传
2020-11-15 上传
2021-12-26 上传

netshell
- 粉丝: 11
- 资源: 185
上传资源 快速赚钱
我的内容管理 展开
我的资源 快来上传第一个资源
我的收益
登录查看自己的收益我的积分 登录查看自己的积分
我的C币 登录后查看C币余额
我的收藏
我的下载
下载帮助

最新资源
- Angular程序高效加载与展示海量Excel数据技巧
- Argos客户端开发流程及Vue配置指南
- 基于源码的PHP Webshell审查工具介绍
- Mina任务部署Rpush教程与实践指南
- 密歇根大学主题新标签页壁纸与多功能扩展
- Golang编程入门:基础代码学习教程
- Aplysia吸引子分析MATLAB代码套件解读
- 程序性竞争问题解决实践指南
- lyra: Rust语言实现的特征提取POC功能
- Chrome扩展:NBA全明星新标签壁纸
- 探索通用Lisp用户空间文件系统clufs_0.7
- dheap: Haxe实现的高效D-ary堆算法
- 利用BladeRF实现简易VNA频率响应分析工具
- 深度解析Amazon SQS在C#中的应用实践
- 正义联盟计划管理系统:udemy-heroes-demo-09
- JavaScript语法jsonpointer替代实现介绍
安全验证
文档复制为VIP权益,开通VIP直接复制
