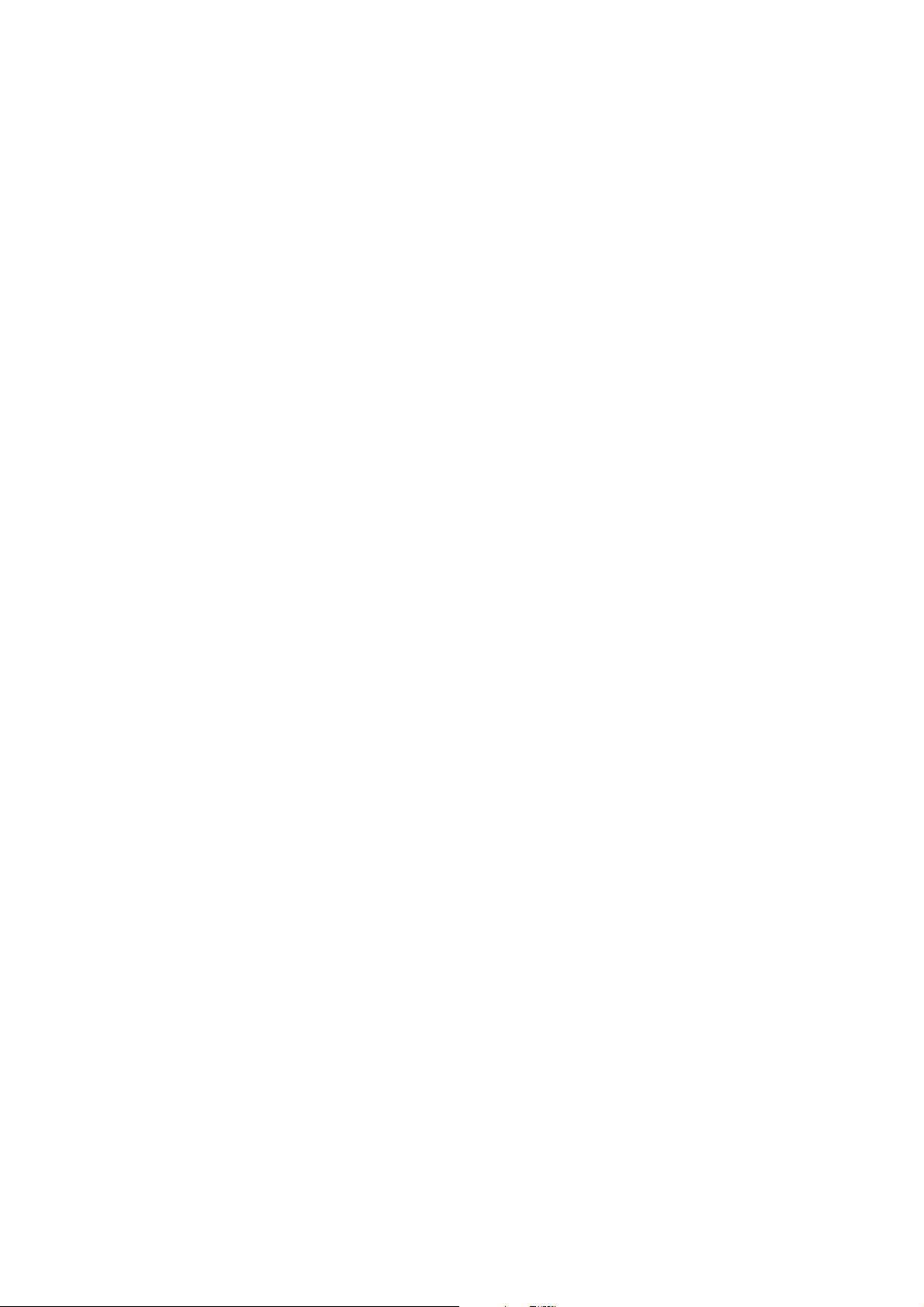
>500MHz), and so many signals are polluted with RF common-mode noise from digital processors
inside their products, that all cables should now be treated as high-frequency.
The three schemes in figure 8 show a hierarchy from a poor system for connecting to a transducer,
through a better one, to a good system. Fitting an A/D converter in the transducer enclosure and
sending high-level encoded data (with error-correction) over the cable to the product for decoding
would be better than the best shown opposite. A perfect system would send the digital data over a
fibre-optic instead of a metallic cable, and such systems are increasingly used in industry.
Concerns about cable shield heating in large or industrial premises are best dealt with by running the
communications cable over a parallel earth conductor (PEC) to divert the majority of the heavy low-
frequency currents (which will prefer to follow paths with lower resistance) and not by ‘lifting’ a shield
connection at one end – which ruins the cable’s shielding benefits at that end. Fitting a capacitor in
series with the shield at one end is also not recommended as a design technique, although it may be
useful as a remedial technique, because it is very difficult to make a capacitive bond work effectively
over the full range of frequencies. PECs and other installation cabling and earthing techniques are
discussed in detail in [2] [3] and [4].
For low frequency signals (say, under 100kHz) higher voltage levels in the communication link are
better, for reasons of immunity. Where signal frequencies are above 10MHz (say) high voltages can
lead to high levels of emissions – lower voltages are often preferred as the best compromise (e.g. as
used by ECL, LVDS, USB). The signal frequency at which lower voltages are preferred depends on
the length of cable and its type and EMC performance (especially its longitudinal conversion loss)
and the design of the transmit and receive circuits.
Transmission line techniques may be essential for high-speed analogue or digital signals, depending
on the length of their connection and the highest frequency to be communicated (see Part 5 of this
series). Even for low-frequency signals, immunity will be improved by using transmission line
techniques for their interconnections.
The best type of cable for EMC usually has a dedicated return conductor associated with each signal
conductor, and any cable shields are used only to control interference. Co-axial cable is generally not
preferred. Some cables need individually shielded signal pairs. It is very important to achieve a good
balance over the whole frequency range, as this means a good common-mode rejection ratio
(CMRR) and hence improved emissions and immunity. Balanced send / receive ICs are good, but
isolation transformers have the benefit of adding galvanic isolation (up to the point where they flash-
over) and also extending the common-mode range well beyond the DC supply rails.
Balanced construction twisted-pair or twinaxial cables usually give the best and most cost-effective
emissions and immunity performance and very small differences in twist (and even the dielectric
constants of the pigments used to colour their insulation) can be important. Balance is so important
that in high-performance circuits even a physically balanced (mirror-image) PCB layout will be
needed, using the same PCB layers.
Transformers and balanced send/receive ICs all suffer from degraded balance at RF. They generally
require a common-mode choke in series to maintain good balance over the whole frequency range of
interest. The CM choke always goes closest to the cable or connector at the boundary of the product.
Transformer isolation, balanced drive and receive, and CM chokes, all help to get the best EMC
performance from a cable.
Figure 9 shows two examples, both equally applicable to providing good emissions and immunity for
digital or analogue signalling (communications) of any speed or frequency range.
Design techniques for EMC – Part1 Cherry Clough Consultants July 2001 Page 19 of 26