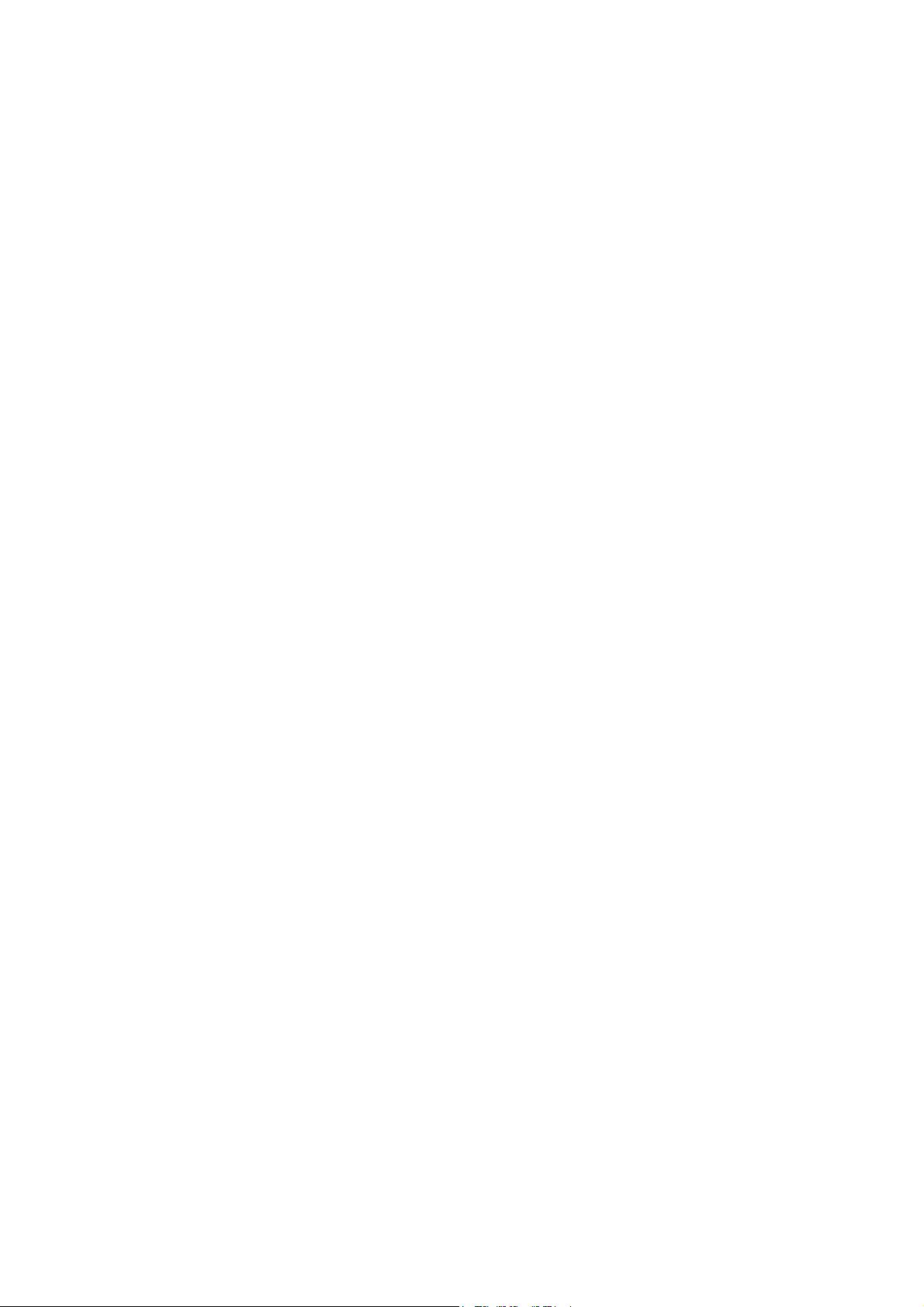
IEEE 802.11ax: Next Generation Wireless Local Area
Networks
Der-Jiunn Deng
1
, Kwang-Cheng Chen
2
, Rung-Shiang Cheng
3
1
Department of Computer Science and Information Engineering, National Changhua University of Education, Taiwan
2
Graduate Institute of Communication Engineering, National Taiwan University, Taiwan
3
Department of Computer and Communication, Kun Shan University, Taiwan
djdeng@cc.ncue.edu.tw, ckc@ntu.edu.tw, rscheng@mail.ksu.edu.tw
Abstract—Recently, IEEE 802 started a task group to investigate
and deliver next generation WLAN technologies for the scenarios
of dense networks with a large number of stations and access
point. The proposal is specified as the IEEE 802.11ax amendment.
Due to the significant network capacity increase achieved by
802.11ax, the term high-efficiency WLAN (HEW) is also used in
reference to this new amendment. This paper summarizes the
IEEE 802.11ax standardization activities in progress and
presents an overview of the most important features proposed in
the 802.11ax amendment. Expected features and challenges for
802.11ax in the design of physical layer (PHY) and media access
control sub-layer (MAC), toward a new era of wireless LANs, are
also discussed.
Keywords-802.11ax, WLANs, GRAP, OFDMA, MU-MIMO,
medium access control, multiple access
I. INTRODUCTION
In past two decades, the IEEE 802.11 wireless local area
networks (WLANs) have experienced tremendous growth with
the proliferation of IEEE 802.11 devices as a major Internet
access for mobile computing. The IEEE standard for WLANs
was initiated in 1988 as IEEE 802.4L, a part of the IEEE 802.4
token bus wired LAN standard, and then change its name to
IEEE 802.11 to form a WLAN standard in 1990. This standard
describes the physical layer (PHY) and medium access control
sub-layer (MAC) specification for wireless connectivity for
fixed, portable and moving stations within a local area. After
delay for many years, IEEE 802.11 working group approved
the draft and later evolving into many amendments, particularly
for higher speed physical layer transmission, namely IEEE
802.11a, 11b, 11g, 11n, 11ac, for enhancement of higher level
service support like 11e (quality of service) and 11i (security),
for wireless access in vehicular environments, 11p, and for
mesh networking , 11s. The list of most popular past and
present 802.11 amendments is shown in Table 1.
A. Beyond 802.11ac
The tremendous growth of mobile data traffic and the
advent of bandwidth-hungry wireless applications highlight the
impending need for large bandwidth and high data rate in next
generation mobile networks. To this end, 802.11ac, the latest
approved 802.11 amendment, has been under development and
has the goal of reaching maximum aggregate network
throughput of at least 1 gigabit per second and a single link
throughput of at least 500 megabits per second on unlicensed
bands at 5 GHz band. This is accomplished by extending the
air interface concepts embraced by 802.11n: wider channel
bandwidth (20/40/80/160/80+80 MHz), more multiple-input
multiple-output (MIMO) spatial streams (up to eight antennas),
and high-density modulation (up to 256-QAM). In particular
downlink multi-user MIMO technology (up to four clients)
adopted improves the spectrum efficiency by allowing
simultaneous transmissions of multiple data frames to different
users. 802.11ac is backward compatible with 802.11a and
802.11n, and the first generation 802.11ac chips are already
available in the market. In contrast to all previous 802.11
amendments, 802.11ac is aimed at improving total network
throughput as well as individual link performance, with
possible integration with cellular systems. The evolution of
WiFi technology is illustrated in Fig. 1.
In the past, the standardization efforts have been very much
focused on increasing the link throughput, rather than efficient
use of spectrum and user experience such as latency.
Nowadays, WLAN devices are currently being deployed in
diverse environments. These environments are characterized by
the existence of many access points (AP) and mobile stations in
geographically limited areas. Increased interference from
neighboring devices and severe collisions from channel
contention in dense environments give rise to network
performance degradation. In addition, WLAN devices are
increasingly required to support a variety of applications such
as voice, video, cloud access, and traffic offloading. While
cellular companies are planning to kick off LTE-A service and
offering up to 1 gigabit per second data rate in the next few
years, WLAN also need to be upgraded to support increasing
demands of network performance by emerging applications,
including improved power consumption for battery-operated
devices, as shown in Fig. 2.
QSHINE 2014, August 18-20, RHODES, Greece
Copyright © 2014 ICST
DOI 10.4108/icst.qshine.2014.256586