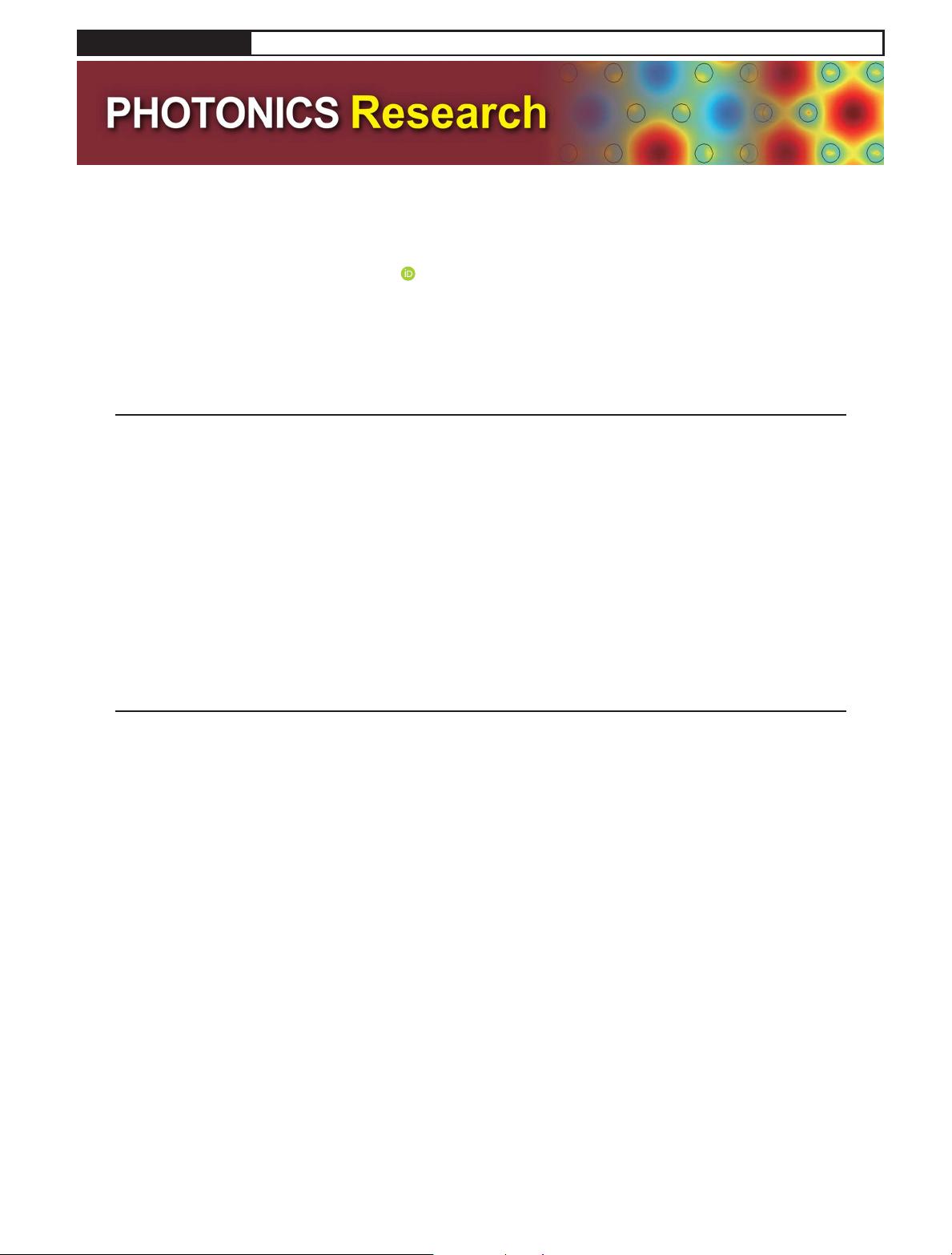
Dark mode plasmonic optical microcavity
biochemical sensor
CHENG LI,
1
LEI CHEN,
1,2
EUAN MCLEOD,
1
AND JUDITH SU
1,3,
*
1
College of Optical Sciences, University of Arizona, Tucson, Arizona 85721, USA
2
State Key Laboratory of Information Photonics and Optical Communications, Beijing University of Posts and Telecommunications,
Beijing 100876, China
3
Department of Biomedical Engineering, University of Arizona, Tucson, Arizona 85721, USA
*Corresponding author: judy@optics.arizona.edu
Received 23 May 2019; revised 23 June 2019; accepted 25 June 2019; posted 25 June 2019 (Doc. ID 368211); published 1 August 2019
Whispering gallery mode (WGM) microtoroid optical resonators have been effectively used to sense low concen-
trations of biomolecules down to the single molecule limit. Optical WGM biochemical sensors such as the microt-
oroid operate by tracking changes in resonant frequency as particles enter the evanescent near field of the resonator.
Previously, gold nanoparticles have been coupled to WGM resonators to increase the magnitude of resonance shifts
via plasmonic enhancement of the electric field. However, this approach results in increased scattering from the
WGM, which degrades its quality (Q) factor, making it less sensitive to extremely small frequency shifts caused
by small molecules or protein conformational changes. Here, we show using simulation that precisely positioned
trimer gold nanostructures generate dark modes that suppress radiation loss and can achieve high > 10
6
Q with an
electric-field intensity enhancement of 4300, which far exceeds that of a single rod (∼2500 times). Through an
overall evaluation of a combined enhancement factor, which includes the Q factor of the system, the sensitivity
of the trimer system was improved 105× versus 84× for a single rod. Further simulations demonstrate that unlike
a single rod system, the trimer is robust to orientation changes and has increased capture area. We also conduct
stability tests to show that small positioning errors do not greatly impact the result.
© 2019 Chinese Laser Press
https://doi.org/10.1364/PRJ.7.000939
1. INTRODUCTION
Whispering gallery mode (WGM) microtoroids [Fig. 1(a)] are
excellent sensors due to their ultra-high-quality (Q) factors and
their ability to be integrated on chip [1–9]. These sensors when
combined with frequency-locking, balanced detection, and
data processing techniques are able to detect wavelength shifts
(< ∼ 0.005 fm) that correspo nd to the detection of a biomol-
ecule a radius of 2 nm binding to the microtoroid [1,2,4].
Coupling plasmonic particles to WGM cavities is one way to
improve the sensitivity of the systems [10]. These particles in-
crease the frequency shift upon molecular binding by providing
enhanced interaction between the optical field and the analyte
due to localized surface plasmon resonance (LSPR) [10,11].
The scattering and absorption losses of the metal, however,
cause degradation of the Q factor of the resonance, that is,
the broadening of the linewidth. Dark mode plasmonic reso-
nances can help solve this problem [12–16], but simulation is
necessary to understand the specific impact of a given plas-
monic structure on the system.
Currently, due to limitations in computing performance and
time consumption, a wedge-shaped model with perfect electric
conductor (PEC) boundary conditions is widely used in plas-
monically enhanced WGM toroid simulations [17]. However,
such boundary conditions act as mirrors, effectively replicating
the plasmonic particle multiple times, which results in an in-
accurate calculation of the coupled system Q factor for a single
particle (see Appendix A for more details).
Here, we use a three-dimensional (3D) eigenmode simula-
tion model of a whole microtoroid with a major diameter of
10 μm to explore the interaction of a single cavity with one
or several nanostructures. The model is implemented in
COMSOL. Due to simulation constraints in both time and
memory, we cannot accurately simulate larger whole toroids
in 3D. The schematic of our 3D model and the field distribu-
tion of the fundamental TE mode are shown in Fig. 1(a). The
polarization of the TE mode is perpendicular to the equatorial
plane of the toroid cavity. Nanorods are placed perpendicularly
to the equatorial plane for maxim um coupling and excitation,
and their near-field enhancement is shown in Fig. 1(c).
We define a figu re of merit known as a combined enhance-
ment factor (f
C
) to quantify the limit of detection and resolution
of our sensing system. With this figure of merit, we can predict
that slightly off-resonant coupling between the plasmon and
WGM provides better performance than a direct match
of the WGM and plasmon resonances. Further, we discuss
the improvements that a lateral dark mode supported by a
Research Article
Vol. 7, No. 8 / August 2019 / Photonics Research 939
2327-9125/19/080939-09 Journal © 2019 Chinese Laser Press