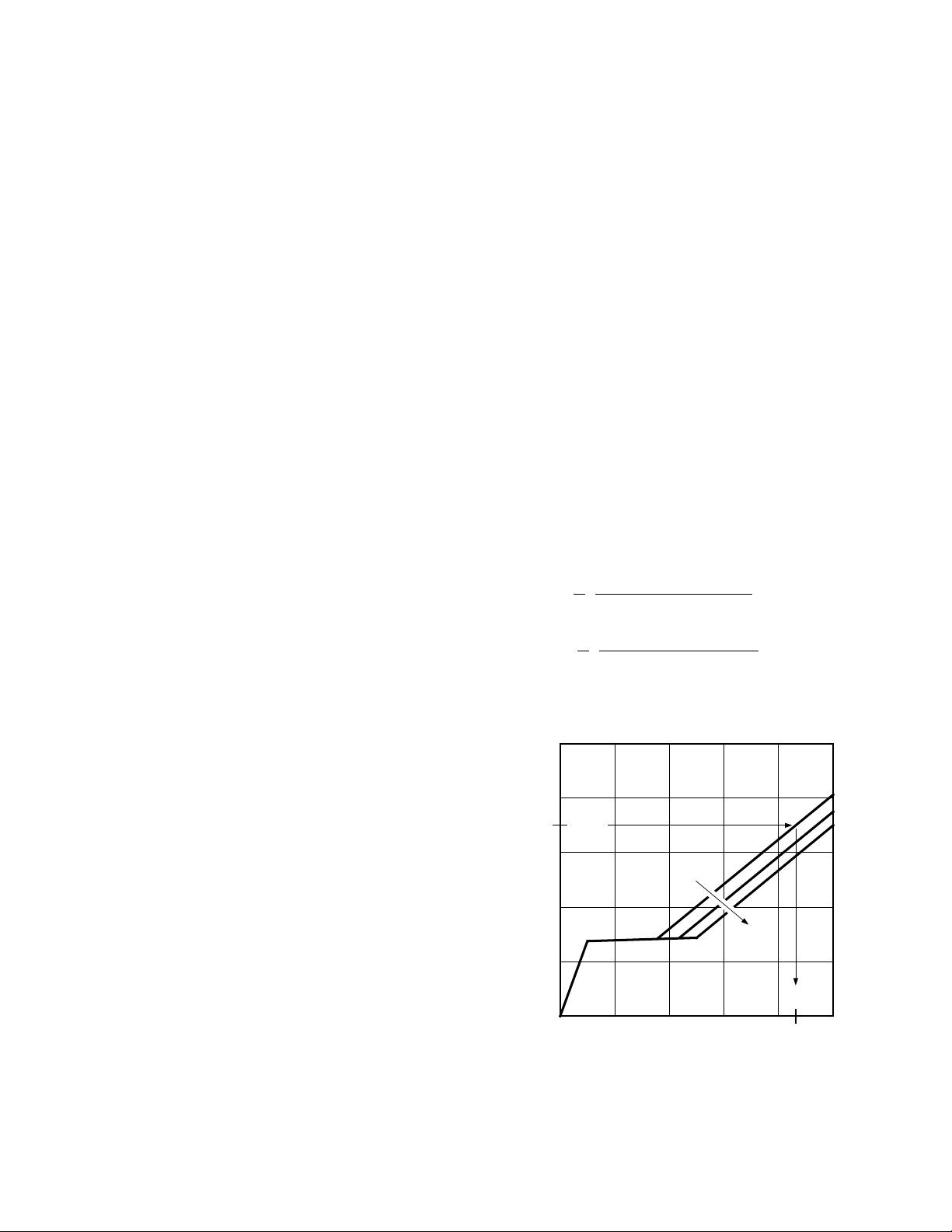
2-8
Summarizing the results, it can be concluded
that the MOSFET transistor can be switched
between its highest and lowest impedance states
(either turn-on or turn-off) in four time intervals.
The lengths of all four time intervals are a
function of the parasitic capacitance values, the
required voltage change across them and the
available gate drive current. This emphasizes the
importance of the proper component selection
and optimum gate drive design for high speed,
high frequency switching applications.
Characteristic numbers for turn-on, turn-off
delays, rise and fall times of the MOSFET
switching waveforms are listed in the transistor
data sheets. Unfortunately, these numbers
correspond to the specific test conditions and to
resistive load, making the comparison of
different manufacturers’ products difficult. Also,
switching performance in practical applications
with clamped inductive load is significantly
different from the numbers given in the data
sheets.
C. Power Losses
The switching action in the MOSFET
transistor in power applications will result in
some unavoidable losses, which can be divided
into two categories.
The simpler of the two loss mechanisms is
the gate drive loss of the device. As described
before, turning-on or off the MOSFET involves
charging or discharging the C
ISS
capacitor. When
the voltage across a capacitor is changing, a
certain amount of charge has to be transferred.
The amount of charge required to change the
gate voltage between 0V and the actual gate
drive voltage V
DRV
, is characterized by the
typical gate charge vs. gate-to-source voltage
curve in the MOSFET datasheet. An example is
shown in Fig. 6.
This graph gives a relatively accurate worst
case estimate of the gate charge as a function of
the gate drive voltage. The parameter used to
generate the individual curves is the drain-to-
source off state voltage of the device. V
DS(off)
influences the Miller charge – the area below the
flat portion of the curves – thus also, the total
gate charge required in a switching cycle. Once
the total gate charge is obtained from Fig. 6, the
gate charge losses can be calculated as:
DRVGDRVGATE
fQVP ⋅⋅=
where V
DRV
is the amplitude of the gate drive
waveform and f
DRV
is the gate drive frequency –
which is in most cases equal to the switching
frequency. It is interesting to notice that the
Q
G
⋅f
DRV
term in the previous equation gives the
average bias current required to drive the gate.
The power lost to drive the gate of the
MOSFET transistor is dissipated in the gate
drive circuitry. Referring back to Figures 4 and
5, the dissipating components can be identified
as the combination of the series ohmic
impedances in the gate drive path. In every
switching cycle the required gate charge has to
pass through the driver output impedances, the
external gate resistor, and the internal gate mesh
resistance. As it turns out, the power dissipation
is independent of how quickly the charge is
delivered through the resistors. Using the resistor
designators from Figures 4 and 5, the driver
power dissipation can be expressed as:
OFFDRV,ONDRV,DRV
IG,GATELO
DRVGDRVLO
OFFDRV,
IG,GATEHI
DRVGDRVHI
ONDRV,
PPP
RRR
fQVR
2
1
P
RRR
fQVR
2
1
P
+=
++
⋅⋅⋅
⋅=
++
⋅⋅⋅
⋅=
Vgs, Gate-to-Source Voltage (V)
Qg, Total Gate Charge (nC)
V
DS
V
DRV
Q
G
Fig. 6. Typical gate charge vs. gate-to-source
voltage.