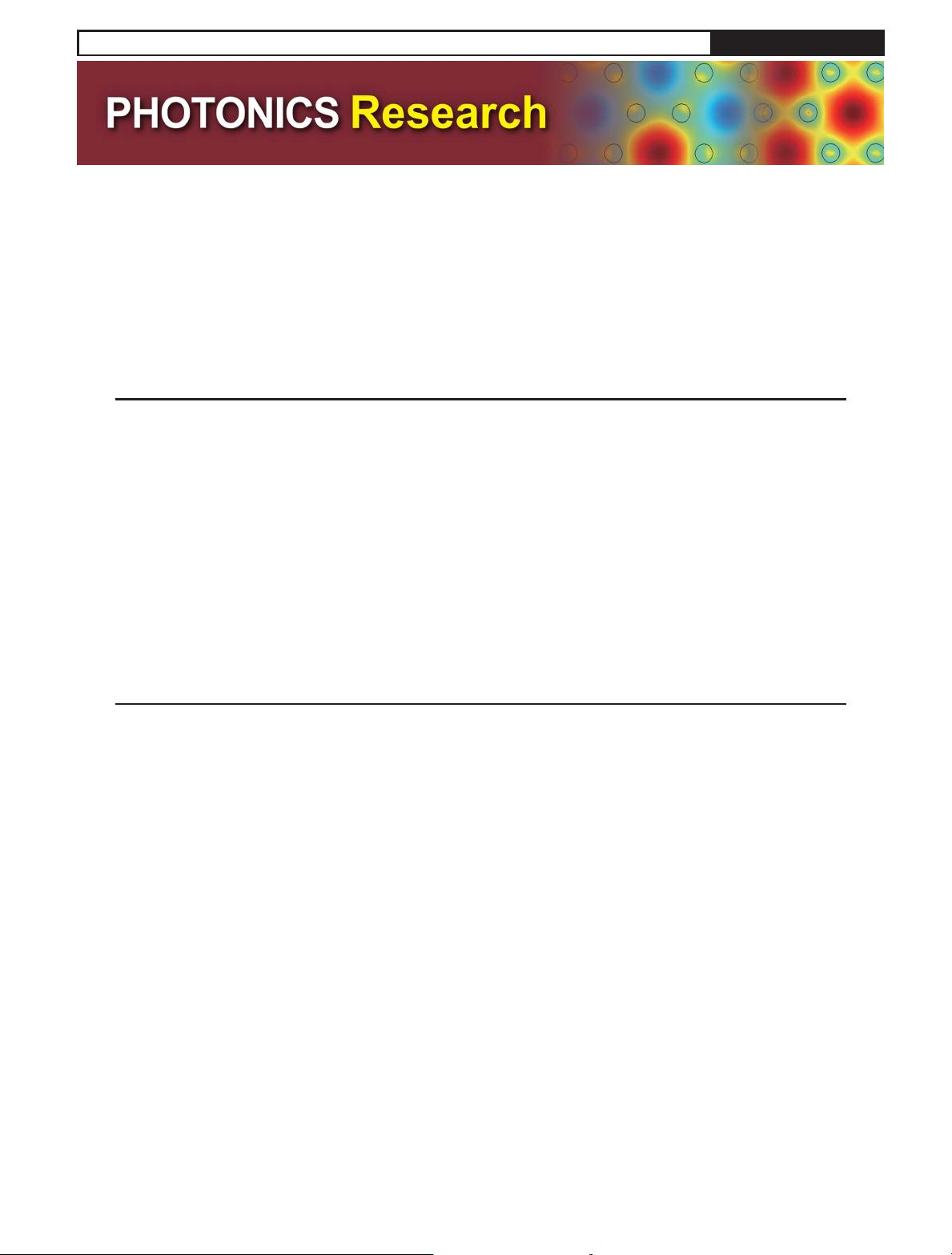
Optical trapping of single quantum dots for
cavity quantum electrodynamics
PENGFEI ZHANG,
1,3
GANG SONG,
1,2
AND LI YU
1
1
School of Science, Beijing University of Posts and Telecommunications, Beijing 100876, China
2
e-mail: songgangbupt@163.com
3
e-mail: pfzhang1980@gmail.com
Received 10 November 2017; revised 28 December 2017; accepted 15 January 2018; posted 17 January 2018 (Doc. ID 313145);
published 27 February 2018
We report here a nanostructure that traps single quantum dots for studying strong cavity-emitter coupling. The
nanostructure is designed with two elliptical holes in a thin silver patch and a slot that connects the holes. This
structure has two functionalities: (1) tweezers for optical trapping; (2) a plasmonic resonant cavity for quantum
electrodynamics. The electromagnetic response of the cavity is calculated by finite-difference time-domain
(FDTD) simulations, and the optical force is characterized based on the Maxwe ll’s stress tensor method. To be
tweezers, this structure tends to trap quantum dots at the edges of its tips where light is significantly confined. To
be a plasmonic cavity, its plasmonic resonant mode interacts strongly with the trapped quantum dots due to the
enhanced electric field. Rabi splitting and anti-crossing phenomena are observed in the calculated scattering spec-
tra, demonstrating that a strong-coup ling regime has been achieved. The method present here prov ides a robust
way to position a single quantum dot in a nanocavity for investigating cavity quantum electrodynamics.
© 2018
Chinese Laser Press
OCIS codes: (350.4855) Optical tweezers or optical manipulation; (240.6680) Surface plasmons; (270.5580) Quantum
electrodynamics.
https://doi.org/10.1364/PRJ.6.000182
1. INTRODUCTION
Strong coupling between a quantum emitter and a cavity
creates a hybridized state that not only attracts interest in
the fundamental study of quantum electrodynamic (QED)
phenomena but also finds a number of applications, such as
quantum information processing [1–3] and ultrafast single-
photon switches [4–6]. The strength of the coupling depends
on the oscillator strength of the emitter as well as the ratio of the
quality factor of the cavity to its mode volume [7]; therefore, a
microcavity with high-Q such as a photonic crystal cavity is
favorable for approaching this regime [8–11]. In recent years,
plasmonic cavities have gained increasing attention, with one
reason being that the localized mode associated with the surface
plasmon polaritons (SPPs) confines the light to a volume scaled
down to cubic nanometers [12–14]. The confined electric field
significantly enhances the strength of the emitter-photon inter-
action. Another reason for the use of a plasmonic cavity is
its ease of integration with other photonic devices for optical
information processing at nanoscales.
Although the small mode volume associated with a micro-
cavity or a plasmonic cavity favors the realization of strong
light–matter coupling, it also presents great challenges for
positioning emitters at the field maximum of the cavity mode
for the strongest interaction. It is especially true when exploring
the QED at single-emitter level [9,10,15–17]. Recently, strong
coupling of single organic dye molecule with plasmonic nano-
cavities was observed by limiting the number of participant
molecules either through host-guest chemistry [15] or by using
the monolayer chain property of J-aggregate [17]. Other tech-
niques of nano-positioning of a single molecule or a cavity for
optimized coupling include scanning probe [18–20], self-
assembly [21], and nanofabrication [8–10]. Besides, the signifi-
cant confinement of light in a microcavity or a plasmonic cavity
produces strong optical gradient force on particles that are
present in the cavity. The optical force then traps the particles
at the antinode or “hot-spot” of the cavity mode for the strong-
est light–matter interaction [22–24]. It is especially interesting
that the presence of the particle itself imposes great influences
on the local electric field and produces a new trapping mecha-
nism, self-induced back-action (SIBA) optical trapping [25].
The active role of the particle enhances the trapping in SIBA
by a magnitude of 1 order and has been used for trapping
dielectric nanoparticles and quantum dots with reduced laser
power [26,27]. Despite this advantage, the previous nanohole
designs for SIBA trapping are not suitable for the study of
strong emitter-cavity coupling.
182
Vol. 6, No. 3 / March 2018 / Photonics Research
Research Article
2327-9125/18/030182-04 Journal © 2018 Chinese Laser Press