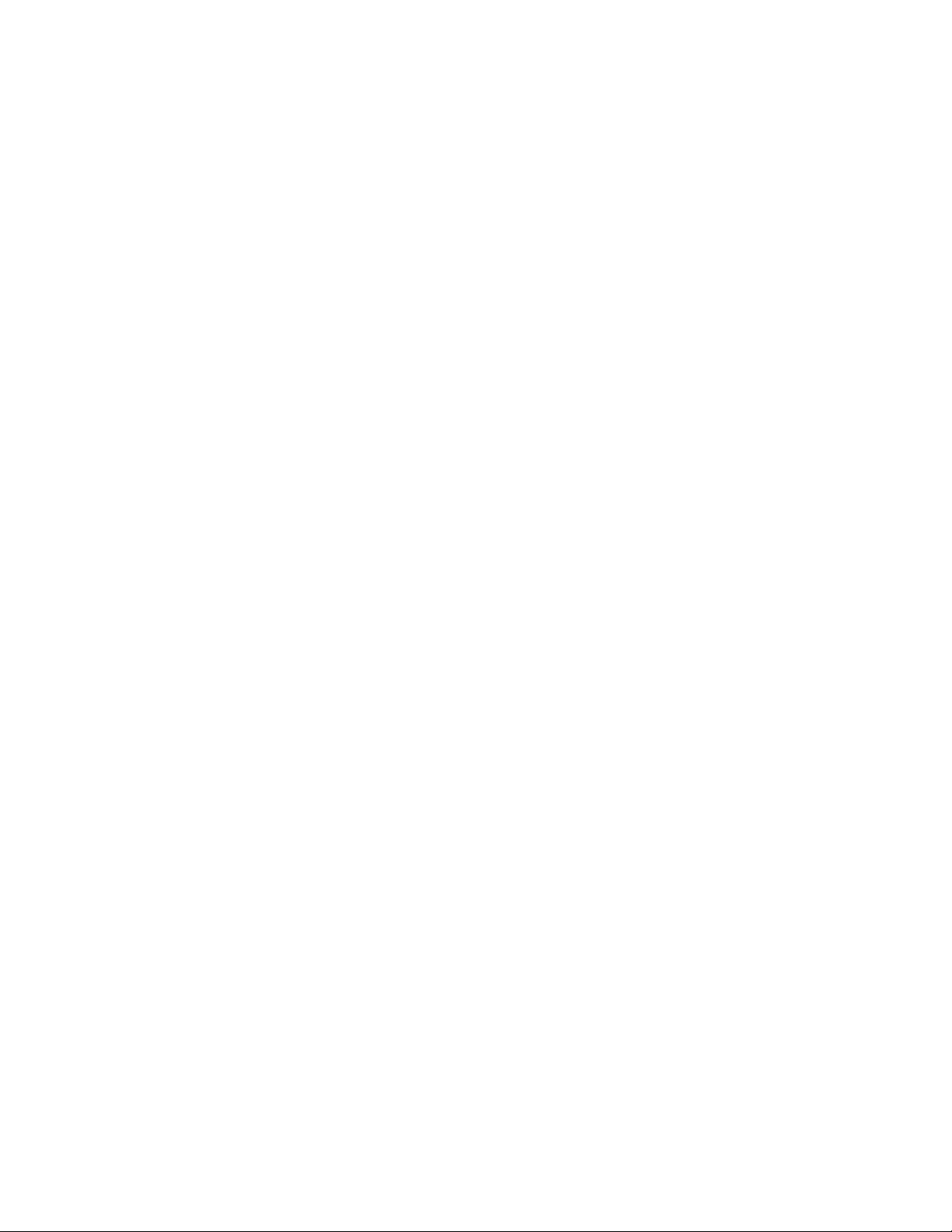
GaAs-based heterojunction photocathodes are nearly optimum candidates in the fabrication of
various optoelectronic devices such as image intensifier tube, polarized electron source, and
solar cell [1–5]. With the development of material growth techniques and the goal of
improving the device performance, extensive efforts based on bandgap engineering have been
made to realize the expected spectral characteristics, and different models and structures have
been proposed in recent years [6–12]. Among them, applying a built-in electric field in the
photocathode interior was proposed to increase collection of generated minority carriers,
which was accomplished by varying the energy band gap [5,6,8,11].
As for various photocathodes in practice, the quantum efficiency is distinctly a very
important parameter utilized to evaluate the performance. The graded band gap in Al
x
Ga
1-x
As
material and Al
x
Ga
1-x
As/GaAs material has been considered previously especially in solar
cells [13–16]. By varying the Al proportion in the Al
x
Ga
1-x
As layer, the bandgap gradation is
achieved, and the built-in electric field is introduced to encourage the transport of excited
photoelectrons to the interface. Moreover, the contact interface recombination action between
Al
x
Ga
1-x
As buffer layer and GaAs emission layer can be reduced and lead to the improved
quantum efficiency. On the other hand, introducing a structure of high-to-low varying doping
concentration from bulk to surface in GaAs emission layer is a novel concept to achieve a
higher photoemission capability [15,17,18]. To sum up, the improvement in the cathode
performance can be attributed to the built-in electric field, which effectively increases the
electron transport efficiency and escape probability.
In view of the function of built-in electric field for photoemitters, the complex Al
x
Ga
1-
x
As/GaAs photocathodes consisting of the composition-graded Al
x
Ga
1-x
As layer and doping-
graded GaAs layer have been grown and activated to negative electron affinity (NEA) state
with the goal of maximizing quantum efficiency [19]. However, it still lacks of proper
quantum efficiency theoretical models for evaluating these complex photocathodes.
Accordingly, how the induced multilevel built-in electric field acts on quantum efficiency is
not clear, and the photoemission mechanism is still not well understood. In this paper, the
theoretical quantum efficiency models for reflection-mode (r-mode) and transmission-mode
(t-mode) Al
x
Ga
1-x
As/GaAs heterostructured photocathodes are deduced by solving from the
one dimensional continuity equations combined with the three-step model [20]. According to
the proposed quantum efficiency models, we explain the superiority in quantum efficiency
over the conventional exponential-doped photocathode, and analyze some related cathode
performance parameters acting on the quantum efficiency characteristics, which would
provide theoretical guidance for the optimum design of graded bandgap photocathodes.
Moreover, in order to verify the theoretical practicality, the r-mode and t-mode graded
bandgap Al
x
Ga
1-x
As/GaAs photocathodes are prepared respectively, and the experimental
quantum efficiency curves are well fitted with the theoretical models.
2. Structure and model
To prevent deterioration of cathode performance arising from lattice mismatch at the interface
between buffer layer and emission layer, and combine the advantages of both composition-
graded and exponential-doped photocathodes, a special structural design regarding the
heterostructured Al
x
Ga
1-x
As/GaAs photocathode is proposed, and the multilayer energy band
structure is shown in Fig. 1. The main difference between r-mode and t-mode photocathodes
is the direction of incident light.
Received 3 Jun 2015; revised 15 Jul 2015; accepted 15 Jul 2015; published 17 Jul 2015
27 Jul 2015 | Vol. 23, No. 15 | DOI:10.1364/OE.23.019478 | OPTICS EXPRESS 19480