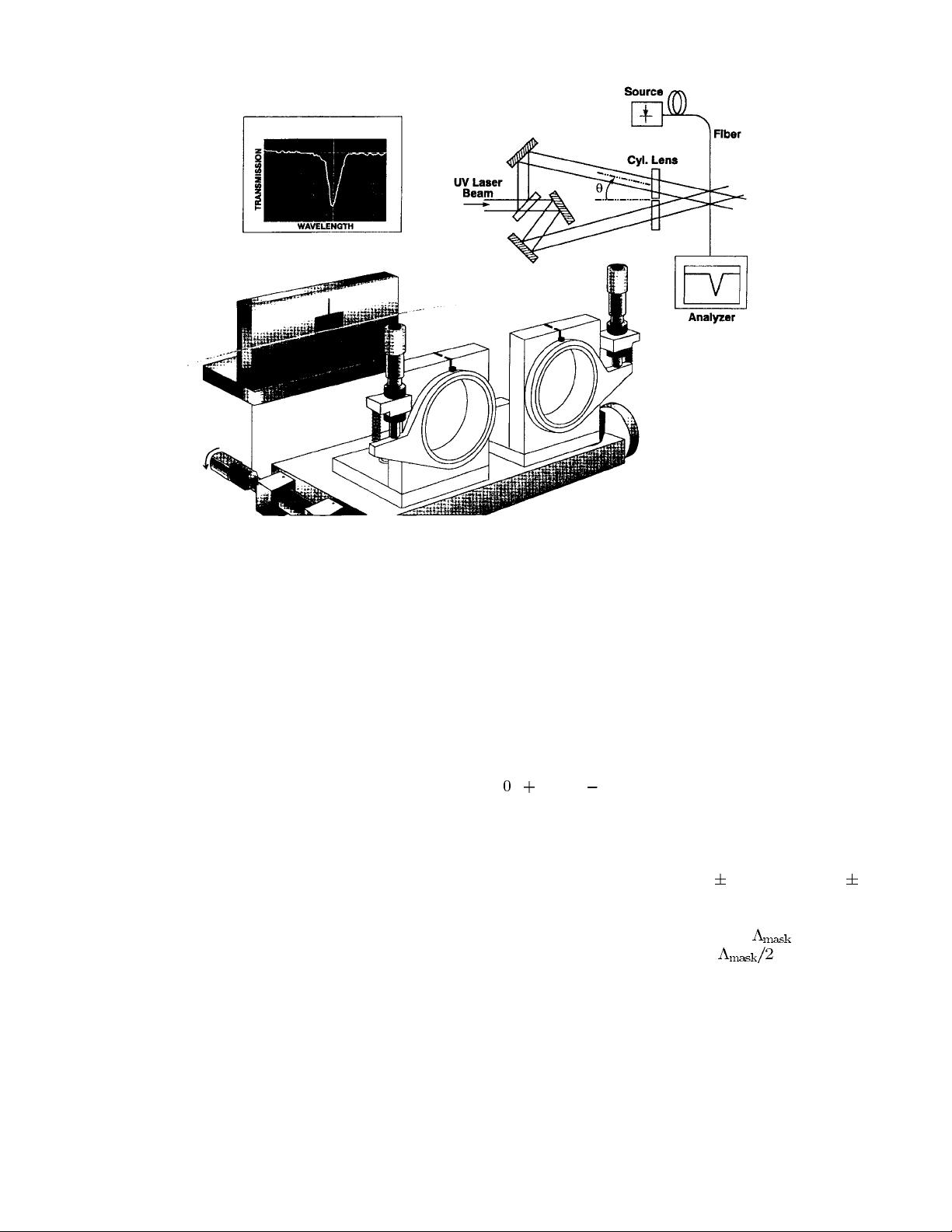
HILL AND MELTZ: FBG TECHNOLOGY FUNDAMENTALS 1265
Fig. 2. Two-beam interferometer arrangement for side-writing fiber Bragg gratings (FBG’s).
enables a single irradiating excimer light pulse to photoin-
duce a large index changes in a small localized region near
the core/cladding boundary. In this case the refractive index
changes are sufficiently large to be observable with a phase
contrast microscope and have the appearance of damaging
physically the fiber. This phenomenon has been used for the
writing of gratings using a single excimer light pulse.
Another property of the photoinduced refractive index
change is anisotropy. This characteristic is most easily
observed by irradiating the fiber from the side with ultraviolet
light polarized perpendicular to the fiber axis. The anisotropy
in the photoinduced refractive index change results in the
fiber becoming birefringence for light propagating through the
fiber. The effect is useful for fabricating polarization mode
converting devices or rocking filters [13].
The physical mechanisms underlying photosensitivity are
not very well understood but are associated with the color
centers in glassy materials. For example, UV photoexcitation
of oxygen-vacancy-defect states [9] in Ge–SiO
2
fiber forms
paramagnetic GeE′ centers that contribute to the index change
[14]. There is also evidence that structural rearrangement of the
glass matrix, possibly densification, is also correlated with the
index increase [15]. The net result of the photoinduced changes
is a permanent change in the refractive index of the glassy
material at wavelengths far removed from the wavelength of
the irradiating ultraviolet light.
IV. G
RATING FABRICATION TECHNIQUES
Historically, Bragg gratings were first fabricated using the
internal writing [1] and the holographic technique [4]. Both
these methods, which have been described already, have been
largely superseded by the phase mask technique [16], [17]
which is illustrated in Fig. 3. The phase mask is made from
flat slab of silica glass which is transparent to ultraviolet light.
On one of the flat surfaces, a one dimensional periodic surface
relief structure is etched using photolithographic techniques.
The shape of the periodic pattern approximates a square wave
in profile. The optical fiber is placed almost in contact with the
corrugations of the phase as shown in Fig. 3. Ultraviolet light
which is incident normal to the phase mask passes through
and is diffracted by the periodic corrugations of the phase
mask. Normally, most of the diffracted light is contained in
the
, 1, and 1 diffracted orders. However, the phase
mask is designed to suppress the diffraction into the zero-
order by controlling the depth of the corrugations in the phase
mask. In practice the amount of light in the zero-order can be
reduced to less than 5% with approximately 40% of the total
light intensity divided equally in the
1 orders. The two 1
diffracted order beams interfere to produce a periodic pattern
that photoimprints a corresponding grating in the optical fiber.
If the period of the phase mask grating is
, the period
of the photoimprinted index grating is
. Note that this
period is independent of the wavelength of ultraviolet light
irradiating the phase mask; however, the corrugation depth
required to obtain reduced zeroth-order light is a function of
the wavelength and the optical dispersion of the silica.
The phase mask technique has the advantage of greatly
simplifying the manufacturing process for Bragg gratings, yet
yielding gratings with a high performance. In comparison with
the holographic technique, the phase mask technique offers
easier alignment of the fiber for photoimprinting, reduced
stability requirements on the photoimprinting apparatus and
lower coherence requirements on the ultraviolet laser beam