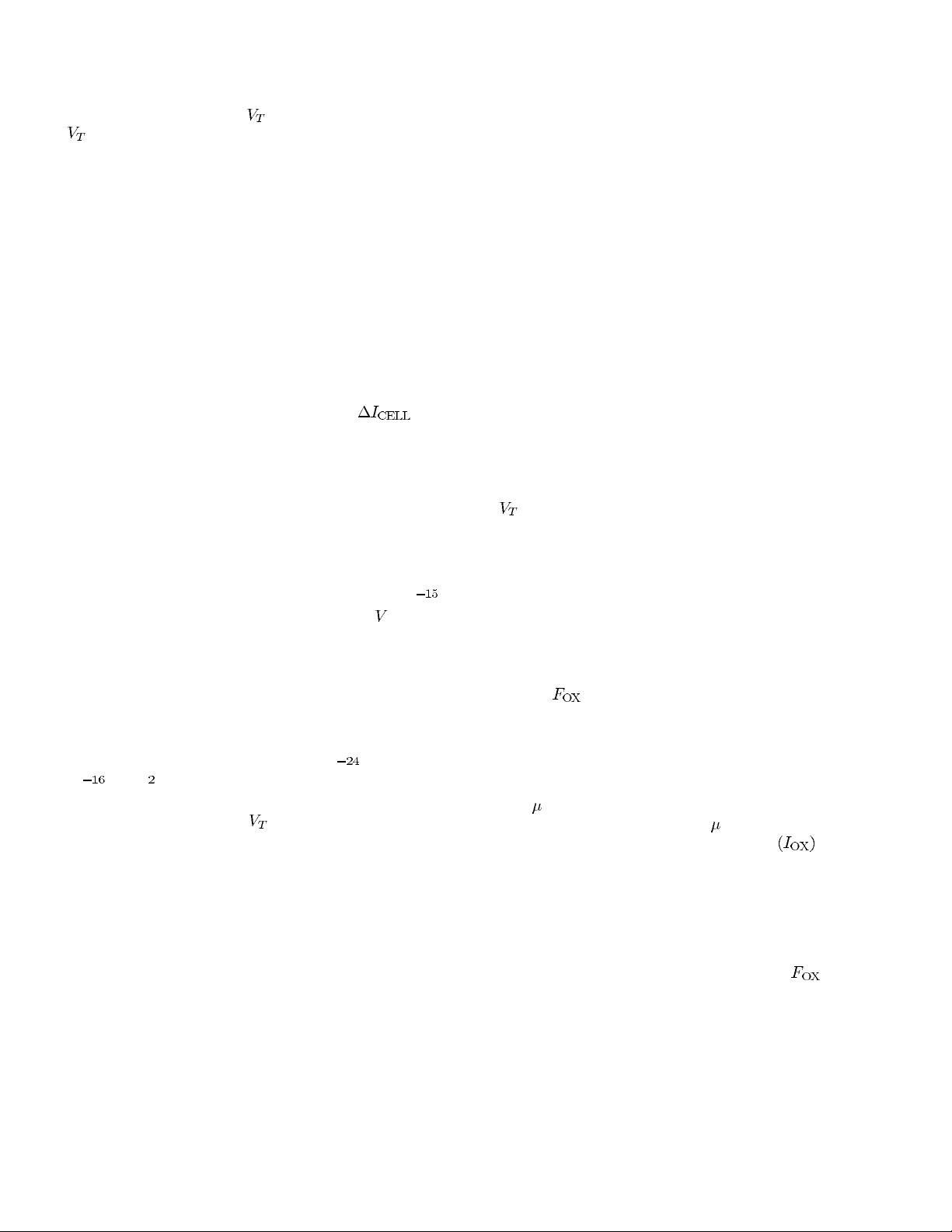
The worst case occurs for the bit-line disturb on a cell
programmed to the highest
level because of the large
shift with respect to the neutral state. Moreover, pro-
gramming the drain voltage is the same as in conventional
Flash memories but the time is longer. On the contrary,
word-line disturb should not be affected substantially by
the increase in program time, at least for a staircase gate-
voltage programming algorithm, since during most of the
time the word-line voltage is rather low and only the
last few pulses will effectively contribute to the disturb.
Optimized cell design for low drain voltage programming
and divided bit-line organization are probably required to
guarantee sufficient program disturb immunity.
As for reading, the operation essentially can be consid-
ered as an analog-to-digital (A/D) conversion of the signal
produced by the addressed cell, and the key issue concerns
the tradeoff between speed and accuracy, in that as the
latter is increased (i.e., as smaller values of
can
be safely recognized) a larger number of bits can be stored
on a single cell, but the sensing circuitry becomes more
expensive and globally slower. In general, the required A/D
conversion can be done in parallel or sequentially for higher
speed and smaller area occupation, respectively, while
intermediate solutions offer interesting tradeoffs between
these conflicting aspects.
With regard to reliability, instead, the crucial problem of
data retention is better expressed in terms of the number
of stored electrons. With FG capacitances in the 10
F range, threshold voltage windows of a few require
variations of about 30 000 electrons in the FG. If this
variation is split, for instance, in four levels, the states of the
sense transistors differ from one another by about 10000
electrons, and this difference should be maintained for more
than ten years. This implies that less than about 1 000
electrons should leak out from (or into) the FG in one year,
i.e., that the (average) leakage currents through the oxide
insulating the FG should be smaller than 10
A (or, about
10
A/cm ). Of course, this problem is significantly
aggravated if the number of levels programmed in a cell
is increased and/or the total
window is narrowed.
The problem can (and must) be alleviated in part with
the use of redundancy- and error-detecting codes, but the
very small numbers given above clearly indicate the need of
outstanding insulating properties. From this point of view,
stress-induced leakage current (SILC), i.e., the excess low-
field conductivity of the oxide induced by the high field
stress used during tunnel programming [22], [23] and/or
oxide time-dependent breakdown poses serious problems.
In any case, MLM’s must meet much more stringent
requirements than conventional bilevel memories in terms
of threshold voltage distribution for each stored level, data
retention, program/read disturb immunity, and design issues
(namely, sense and program accuracies) [21], [24], [25].
D. Problem Interaction
The successful realization of MLM’s and the number
of bits that can be stored in a single cell depend on the
solutions given to the main problems mentioned above
(accurate charge placement, cell sensing, and reliability).
Such solutions, however, are strongly dependent on the
physical mechanism used for cell writing as well as on
the memory architectures, which are fundamental ingre-
dients determining the complexity, performance, and cost
effectiveness of memory chips.
Thus, the realization of MLM’s represents a unique
global problem composed of many interacting aspects that
must be considered concurrently in the conception and
development of real products, as well as in the analysis
of the subject, as is done in the rest of this paper.
II. B
ASICS IN NV MEMORIES
A. Writing Mechanisms
MLM’s make use of the same physical mechanisms
for injecting and/or extracting electrons from the FG as
their conventional (i.e., bilevel) counterparts, namely CHE
injection and FN tunneling, while UV exposure can also
be used for erasing. ML operation, however, has more
stringent requirements since it needs accurate control of
the
distributions of the different levels, which must not
only be narrow but also well separated.
CHE injection and FN tunneling present specific features
that interact strongly with circuit and architectural aspects;
these are briefly reviewed below.
The CHE mechanism enables electrons to be injected
into the cell FG by applying a suitable medium–high drain
voltage (to heat the electrons within the transistor channel)
and a high gate voltage, providing both adequate electron
density in the channel and sufficient electric field in the
gate oxide (
, in any case significantly lower than that
required for FN tunneling).
The injection efficiency of CHE injection is very small,
as the current flowing through the gate oxide is only a very
small fraction of the cell-drain current. Typical values of
drain currents during programming are in the range of a few
hundred
A per cell, although an optimized cell structure
can operate with currents around 100
A or less [26], while
the average current injected into the gate oxide
is in
the range of 0.1 to 1 nA.
Naturally, it is not possible to heat electrons within the
FG by means of an electric field; thus the hot electron
mechanism cannot be used to extract electrons from it.
FN tunneling, instead, allows injection of cold carriers
and gives rise to a current flowing into (or from) the FG,
that exhibits a (quasi) exponential dependence on
and
can be reversed by simply changing the sign of such a
field; thus it can be used for both injection and extraction
of electrons into/from the FG. For these operations to be
completed in practical times, a very high field is needed (in
the range of 8–10 MV/cm), which gives rise to reliability
problems ultimately limiting the writing speed, while the
high voltages required to generate adequate fields are not
easy to handle at the circuit level.
Two different FN tunneling schemes are of interest for
NV memories. In the first one (channel FN tunneling),
RICC
`
O et al.: NONVOLATILE MULTILEVEL MEMORIES 2403