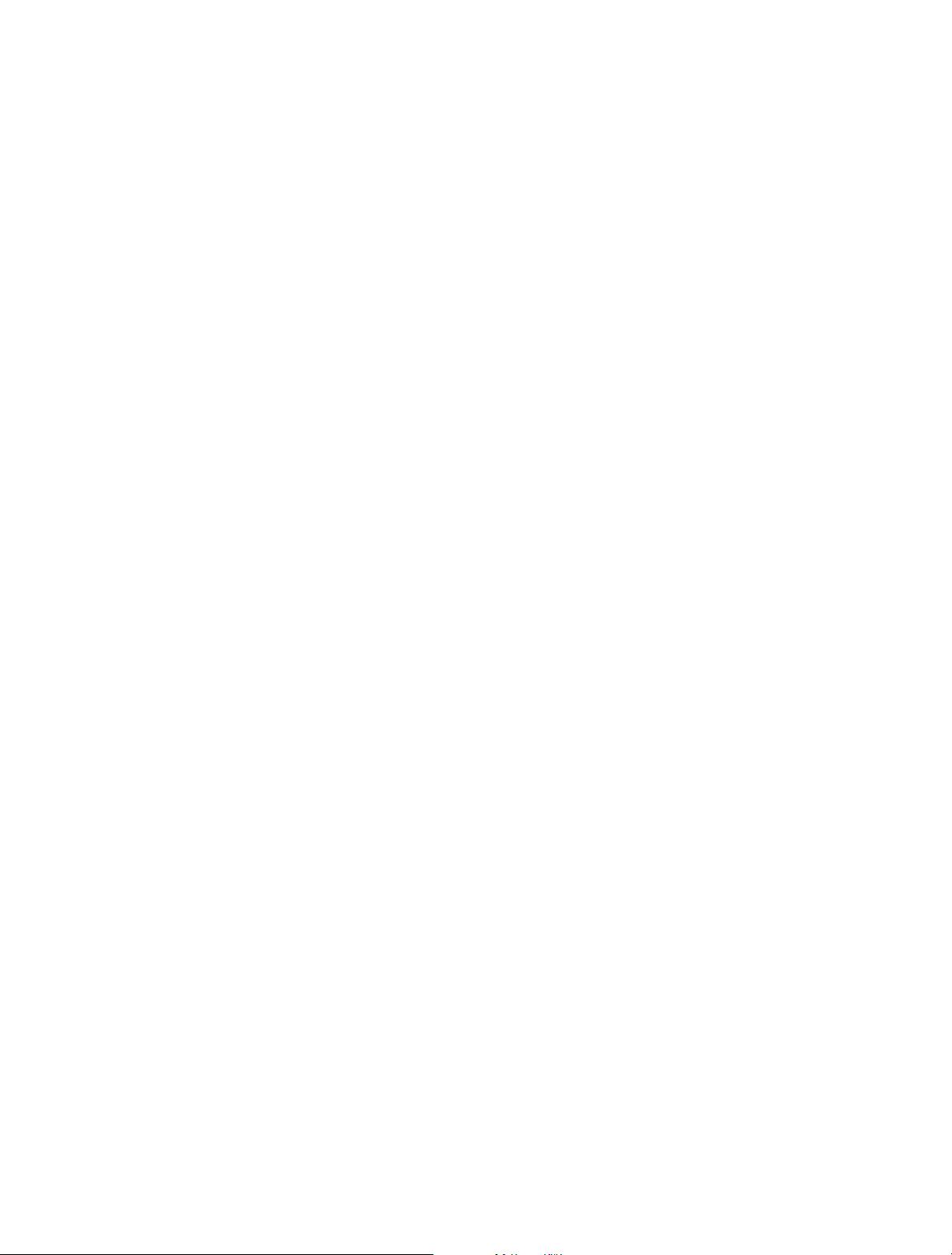
Deep-UV fluorescence lifetime imaging microscopy
Christiaan J. de Jong,
1,2,†
Alireza Lajevardipour,
1,†
Mindaugas Gecevičius,
3,†
Martynas Beresna,
3,†
Gediminas Gervinskas,
1,4,†
Peter G. Kazansky,
3,†
Yves Bellouard,
5,†
Andrew H. A. Clayton,
1,†
and Saulius Juodkazis
1,4,6,
*
,†
1
Centre for Micro-Photonics, Faculty of Science, Engineering and Technology, Swinburne University of Technology,
Hawthorn, VIC 3122, Australia
2
Department of Mechanical Engineering, Eindhoven University of Technology,
Postbus 513, 5600MB Eindhoven, The Netherlands
3
Optoelectronics Research Centre, University of Southampton, Southampton, SO17 1BJ, UK
4
Melbourne Centre for Nanofabrication, 151 Wellington Road, Clayton, VIC 3168, Australia
5
Ecole Polytechnique Fédérale de Lausanne, Rue de la Maladiére 71b, CH—2002 Neuchâtel, Switzerland
6
Center for Nanotechnology, King Abdulaziz University, Jeddah 21589, Saudi Arabia
*Corresponding author: sjuodkazis@swin.edu.au
Received July 2, 2015; revised August 21, 2015; accepted August 22, 2015;
posted August 24, 2015 (Doc. ID 244203); published September 28, 2015
A novel fluorescence lifetime imaging microscopy (FLIM) working with deep UV 240–280 nm wavelength excita-
tions has been developed. UV-FLIM is used for measurement of defect-related fluorescence and its changes upon
annealing from femtosecond laser-induced modifications in fused silica. This FLIM technique can be used with
microfluidic and biosamples to characterize temporal characteristics of fluorescence upon UV excitation, a capabil-
ity easily added to a standard microscope-based FLIM. UV-FLIM was tested to show annealing of the defects induced
by silica structuring with ultrashort laser pulses. Frequency-domain fluorescence measurements were converted into
the time domain to extract long fluorescence lifetimes from defects in silica. © 2015 Chinese Laser Press
OCIS codes: (110.0180) Microscopy; (260.7190) Ultraviolet; (350.3390) Laser materials processing.
http://dx.doi.org/10.1364/PRJ.3.000283
1. INTRODUCTION
Femtosecond laser direct writing is a powerful technique
with applications ranging from optofluidics and optome-
chanics [1–3] to birefringent optical elements [4] and optical
data storage [5]. Under certain experimental conditions,
femtosecond laser irradiation can induce self-assembled
nanostructure in the bulk of transparent material [6–8] or im-
print complex polarization fields onto a surface [9–11]. The
subwavelength arrangement of this modification results in
optical anisotropy (the form birefringence), which provides
control over polarization of traversing light; hence, polariza-
tion controlling optical elements can be made using nanograt-
ings. The nanogratings are observed only in a handful of
materials such as sapphire, tellurium oxide, germanium oxide,
and silica glasses. The mechanisms of nanograting and
surface ripple formation are closely related and are actively
researched (for a recent review [11]). The bulk ripples are
formed at subcritical free carrier plasma densities; the break-
down occurs at the permittivity ε
0
0 (real part) when the
critical plasma density is reached. At a higher excitation
and over-critical plasma densities when ε
0
≤ −1 a surface
wave (the surface plasmon polariton) can be formed, which
is imprinted onto the surface by ablation [11]. In both cases of
the bulk and surface ripples, the period is closely following
Λ λ
0
∕nI∕2, where the refractive index n is intensity, I,
dependent, and λ
0
is the light wavelength in vacuum [11].
Formation of the nanostructure in dielectrics is accompa-
nied by the rearrangement of chemical bonds and formation
of optically, magnetically, and electrically active defects.
Some of them, under UV irradiation, emit strong fluorescence,
which is detectable from the laser-structured areas that can
strongly impede imaging and fluorescence lifetime measure-
ments popular in bio and medical research. The fluorescence
lifetime imaging microscopy (FLIM) has become a popular
technique in cell imaging and is used for investigation of en-
ergy transfer mechanisms and can deliver a super-resolution
capability [12–14]. Addition of UV functionality to FLIM is an
appealing direction due to a possibility of direct excitation of
proteins [15] without their labeling. Further insight into ger-
micidal properties of deep-UV (240–280 nm) light [16–18]
could be achieved with UV-FLIM. LEDs are used in FLIM,
however, not in deep UV range [19].
Here, we show the UV capability, which can be added to
standard microscope-based FLIM setups. This UV-FLIM func-
tionality is demonstrated by fluorescence analysis of laser-
induced defects in silica where polarization converters were
formed by fs-laser writing. It is shown that annealing at
300°C for 2 h fully removes the fluorescence at 650 nm
and longer wavelengths without suppressing the form
birefringence required for polarization conversion. The UV-
fluorescence-lifetime-imaging method revealed the removal
of long-time relaxation components from the fluorescence
time decay in the defect-fluorescence band in silica. This ex-
pands use of fluorescence under UV excitation demonstrated
for recognition of protein crystals [20].
2. METHOD
The frequency domain realization of FLIM is based on the tem-
poral modulation of the excitation and on the synchronized
detection of the phase-shifted signal [12–14]. To characterize
de Jong et al. Vol. 3, No. 5 / October 2015 / Photon. Res. 283
2327-9125/15/050283-06 © 2015 Chinese Laser Press