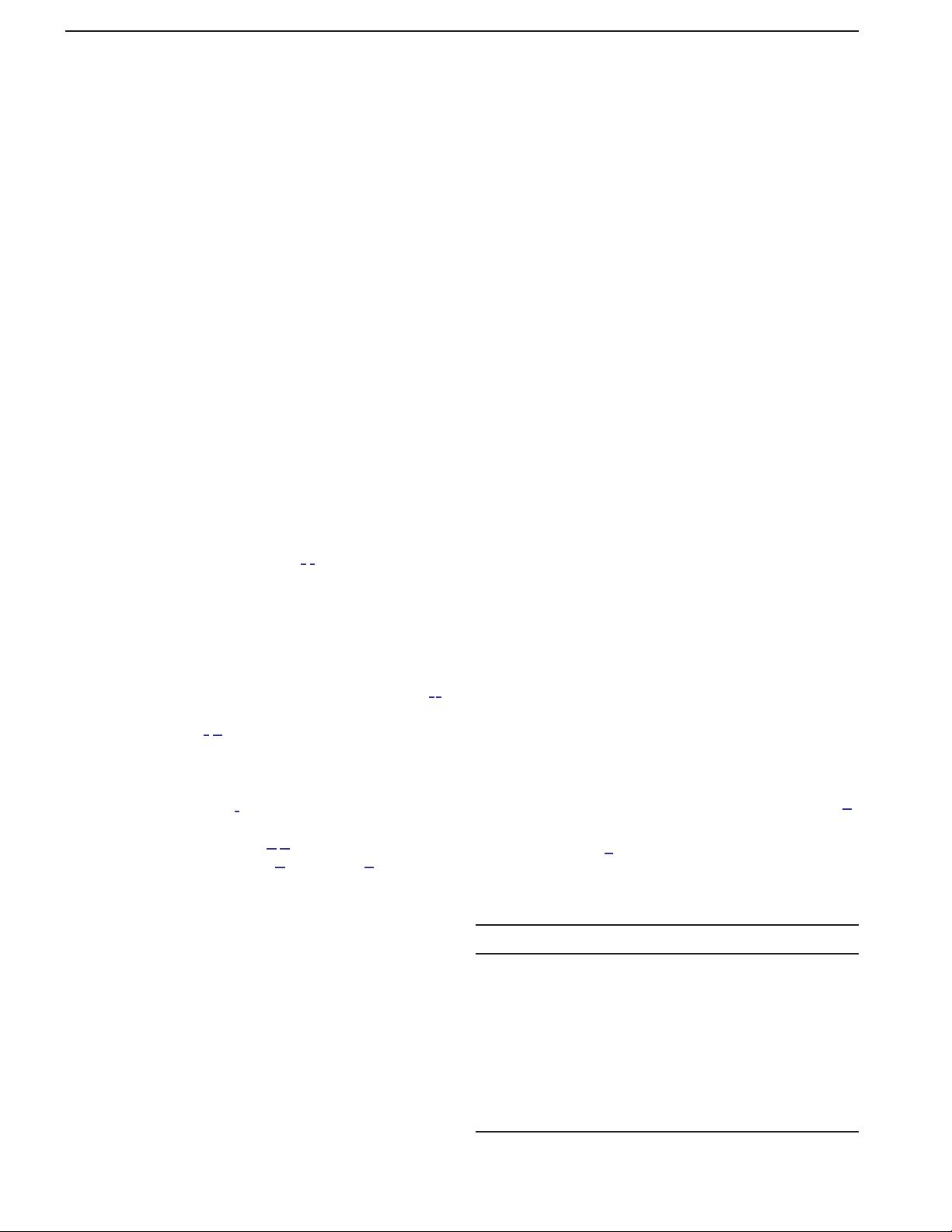
Laser diode pumped high-energy single-frequency Er:YAG
laser with hundreds of nanoseconds pulse duration
Shanghua Li (李尚桦)
1,2
, Qing Wang (王 庆)
1,2,
*, Rui Song (宋 睿)
1,2
,
Fangfang Hou (侯方方)
1,2
, Mingwei Gao (高明伟)
1,2
, and Chunqing Gao (高春清)
1,2
1
School of Optics and Photonics, Beijing Institute of Technology, Beijing 100081, China
2
Key Laboratory of Information Technology, Ministry of Industry and Information Technology,
Beijing Institute of Technology, Beijing 100081, China
*Corresponding author: qingwang@bit.edu.cn
Received October 27, 2019; accepted November 22, 2019; posted online February 18, 2020
We demonstrated a high-energy single-frequency erbium-doped yttrium aluminum garnet (Er:YAG) laser. With
1470 nm laser diodes (LDs) as pumping sources, single-frequency laser pulses with energy of 28.6 mJ, 21.6 mJ,
and 15.0 mJ are obtained at pulse repetition frequency of 200 Hz, 300 Hz, and 500 Hz, respectively. As far as we
know, this is the highest single-frequency pulse energy with the Er:YAG gain medium. With the ring cavity
design, pulse duration is maintained at hundreds of nanoseconds. This high-energy single-frequency laser with
hundreds of nanoseconds pulse duration is a prospective laser source for light detection and ranging applications.
Keywords: Er:YAG; injection-locking; single-frequency; high energy.
doi: 10.3788/COL202018.031401.
A single-frequency erbium-doped yttrium aluminum gar-
net (Er:YAG) laser operating at an eye-safe wavelength
has drawn much attention in various applications like
plastics processing, laser medicine, and wind measurement
light detection and ranging (Lidar)
[1–5]
. For coherent wind
measurement Lidar, range and radial velocity of aerosols
need to be measured at the same time. For long range de-
tection, a high-energy single-frequency laser is required ,
and for accurate radial velocity detection, single-frequency
laser pulses with longer pulse duration are required. So, in
coherent wind measurement Lidar systems, laser pulses
with duration of hundreds of nanoseconds are used
[6,7]
.
There are plenty of works on such a kind of single-fre-
quency laser system
[8–13]
. Most of the previous works on
a single-frequency pulsed laser are based on a single gain
medium, and the highest pulse energy with a single gain
medium at 1645 nm is 16 mJ, with a pulse repetition fre-
quency (PRF) of 250 Hz
[6]
.
For higher energy, a double gain-medium configuration
has been used in recent years
[14–17]
. Single-frequency laser
pulses with energies of 20.9 mJ
[15]
and 21 mJ
[16]
have been
obtained at a PRF of 200 Hz and 500 Hz, with pulse du-
ration of 110 ns and 93 ns, respectively.
These double gain-medium high-energy lasers produce
laser pulses with duration around 100 ns, while wind Lidar
application requires laser pulses with a duration of hun-
dreds of nanoseconds. Furthermore, for longer detection
range, a higher-energy laser pulse is needed and will lead
to shorter pulse duration, which will deteriorate the Lidar
performance.
Besides, these single-frequency Er:YAG la sers are com-
pletely or partially pumped by a 1532 nm fiber laser, with
an extra power transfer stage from a laser diode (LD) to an
Er,Yb co-doped fiber laser, resulting in lower electrical to
optical efficiency and a complicated system.
In this Letter, we demonstrate an LD pumped high-
energy single-frequency Er:YAG laser at 1645 nm, with
the pulse duration maintaining hundreds of nanoseconds.
For high-energy laser pulses generation, we compared the
common in-band pump source of a 1532 nm LD and
1470 nm LD theoretically. The analysis indicates that the
1470 nm LD outperforms the 1532 nm LD under high-
energy operation. By using two 1470 nm LDs as pump
sources and injection-locking technology, single-frequency
laser pulses with pulse energy of 28.6 mJ, 21.6 mJ, and
15.0 mJ are obtained at PRFs of 200 Hz, 300 Hz, and
500 Hz. The pulse duration is maintained at 159 ns, 191 ns,
and 263 ns, respectively, whi ch satisfies the requirement of
wind Lidar.
The center wavelengths of the in-band pump LD for the
Er:YAG system are 1470 nm and 1532 nm, respe ctively. A
simple model is used to compare the maximum upper-
state density under different pumping wavelengths
[18]
.
The definitions of the parameters used in this Letter
are listed in Table
1.
Table 1. Definition of the Parameters
Item Definition
ΔN Inversion density
f
2
Pump transition upper-state Boltzmann factor
f
1
Pump transition lower-state Boltzmann factor
N
2
Upper-state population density
N
1
Lower-state population density
N Total dopant concentration
β Normalized upper-state density
COL 18(3), 031401(2020) CHINESE OPTICS LETTERS March 2020
1671-7694/2020/031401(5) 031401-1 © 2020 Chinese Optics Letters