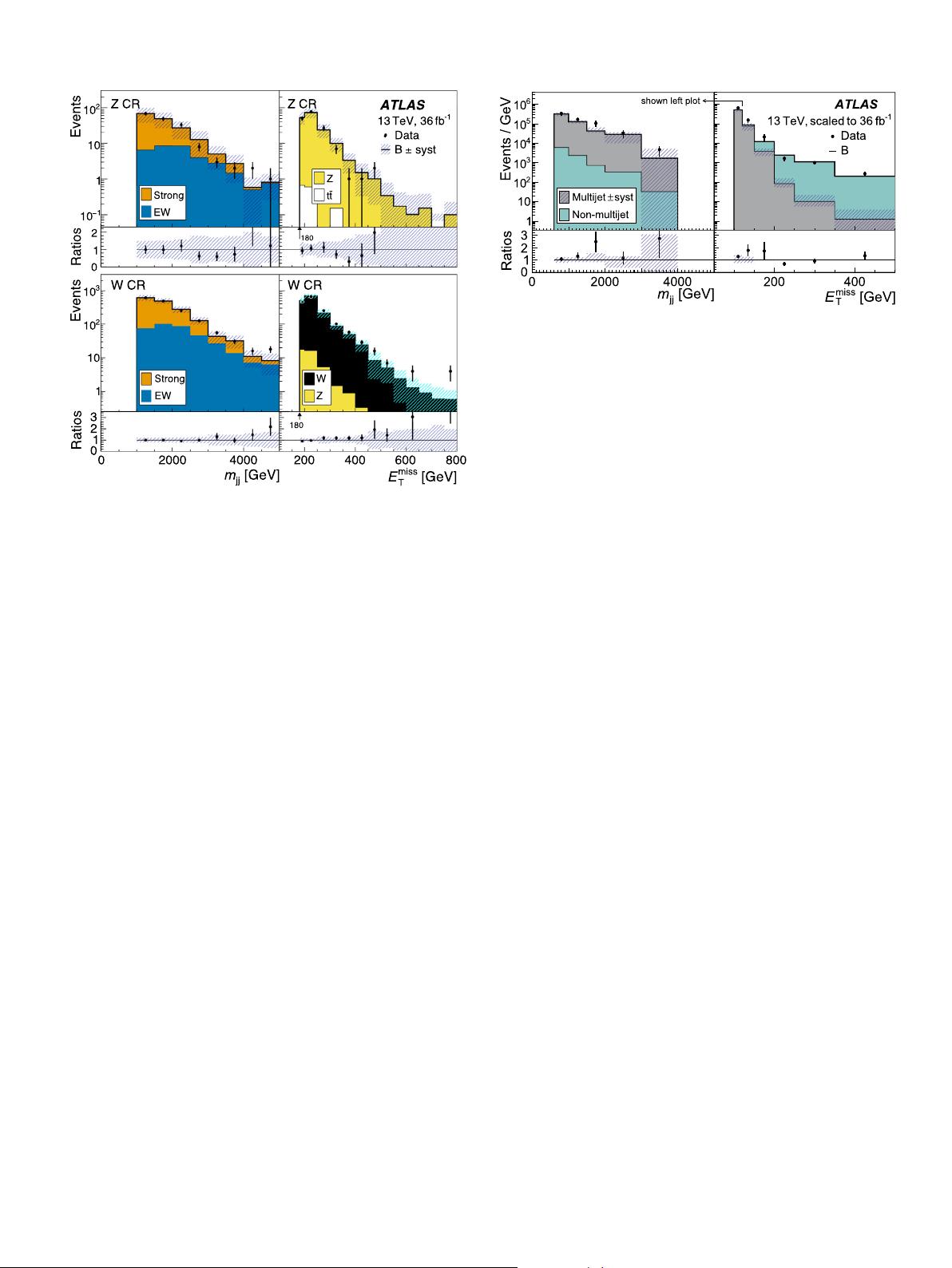
The ATLAS Collaboration / Physics Letters B 793 (2019) 499–519 503
Fig. 2. Distribution of event yields in the Z (top) and W (bottom) control regions.
The postfit normalizations for m
jj
(left) and E
miss
T
(right) are summed over the sub-
samples.
The E
miss
T
distributions start at 180 GeV as indicated. The observed data N
(dots)
are superimposed on the sum of the backgrounds B (stacked histogram with
shaded systematic uncertainty bands). The breakdown of the B is given in the lower
left box in each panel. The bottom panels show the ratios of N to B with the sys-
tematic
uncertainty band shown on the line at 1. The “other,” as listed in Table 1,
contribute a few events at low values of m
jj
and E
miss
T
, and are omitted. The last
bin in each plot contains the overflow.
The postfit value of ν
fake
(the product ρ
fake
·ν
fake
) is the abso-
lute
number of e fake events in the W
high
e
ν
(W
low
e
ν
) subsamples.
Since there is a ν
fake
parameter for each bin i, the m
jj
shape is
also predicted. Apart from determining the ρ
fake
value, which is
fixed in the fit, F
eν
is not part of the fit model. We note that the
W
high
e
ν
-W
low
e
ν
samples are split by charge, because W
±
production
is not symmetric in pp collisions. However, the same ν
fake
param-
eter
is used for both charges because the e fakes are expected to
be symmetric in charge since they originate mostly from multijet
events.
The remaining processes—top quarks, dibosons, multijets—
contribute
negligibly to the SR (called “other” in Table 1). The
first two are estimated with MC using nominal cross sections. The
multijet contribution is very small, but it is a difficult process to
estimate. It is a potentially dangerous background because those
events that pass the E
miss
T
selection are mostly due to instrumen-
tal
effects.
The billionfold-or-more reduction of multijets after the event
selection makes it impractical to simulate, so a data-driven method
based on a rebalance-and-smear strategy [72]is used. The assump-
tion
is that the E
miss
T
is due to jet mismeasurement in the detector
response to jets and neutrinos from heavy-flavor decays [73,74].
Using the jet-triggered sample, the jet momenta are rebalanced by
a kinematic fit, within their experimental uncertainties, to obtain
the balanced value of the jets’ p
T
. The rebalanced jets are smeared
according to jet response templates, which are obtained from MC
and validated with dijet data. The rebalance-and-smear method
predicts both the shape of the E
miss
T
distribution and the absolute
normalization. The procedure is verified in a φ
jj
-sideband vali-
dation
region (VR) with 95% purity of QCD multijet events. This
VR is defined by 1.8 < | φ
jj
|< 2.7 and the loosening of the other
requirements (| η
jj
|> 3, m
jj
> 0.6 TeV, and allow a third leading
jet with 25 < p
T
< 50 GeV, but no other jets with p
T
> 25 GeV). The
Fig. 3. Distribution of event yields in the multijet validation region for m
jj
(left) and
E
miss
T
(right). The m
jj
plot shows the 100< E
miss
T
< 120 GeV subset of the right plot
as indicated by the arrow. The N observed data (dots) are superimposed on the
sum of the B backgrounds (stacked histogram). The systematic uncertainty band
applies only to the multijet component. The statistical uncertainties are relatively
large because of the weighting of the trigger samples with large prescale values.
See the caption of Fig. 2 for other plotting details.
comparison of the predictions and the data in the VR shows good
agreement (Fig. 3). The multijet component is obtained using the
rebalance-and-smear method with the associated systematic un-
certainty
bands, while the non-multijet components are obtained
using MC.
6. Uncertainties
Experimental and theoretical sources of uncertainties as well as
the correlations between the various sources are described. The re-
sulting
impact of the uncertainties on the yields and on the signal
sensitivity is summarized later in Table 2.
Experimental sources of uncertainty are due mainly to the jet
energy scale and resolution [75], E
miss
T
soft term [76], and lep-
ton
measurements [42,43]. In order to reduce fluctuations due to
limited MC sample size, the uncertainties in number of expected
events for the variations of jet energy scale and resolution for the
strong and electroweak background samples are averaged. This is
motivated by the similarities of the kinematics and the detector ef-
fects
for the two production processes for each m
jj
bin. The uncer-
tainty
related to lepton identification or veto has a non-negligible
(negligible) effect on α
W
(α
Z
) because of the following scenarios.
The W
ν
background is significant in the SR, which results in an
uncertainty for the cases related to the lepton veto. The Z
back-
ground
is negligible in the SR, because the selection requires there
to be no leptons.
The following experimental sources have small or negligible
impact in the final result. The pileup distribution and luminosity
[77,78]have a relatively small impact. The trigger efficiency mod-
eling,
for both the lepton triggers for the CR and E
miss
T
triggers for
the SR, are not listed in Table 2. Their impact on the events yields
was at the 1% level and their impact on the signal sensitivity are
found to be negligible.
Theoretical sources of uncertainty are due mainly to scale
choices in fixed-order matrix-element calculations. For the back-
ground
processes, QCD scales are varied for the resummation
scale (resum.), renormalization scale (renorm.), factorization scale
(fact.), and ckkw matching scale. The first three scales in the list—
technically
called q
2
, μ
R
, μ
F
, respectively—are varied by a factor
of two [79,80]. For the ckkw matching scale between the matrix
element and the parton shower [60], the central value and the
considered variations are 20
+10
−5
GeV. The higher-order electroweak
corrections to the strongly produced W or Z are found to be neg-
ligible.