没有合适的资源?快使用搜索试试~ 我知道了~
首页斯坦福大学Andrea Goldsmith教授的无线通信指南
斯坦福大学Andrea Goldsmith教授的无线通信指南
需积分: 10 4 下载量 178 浏览量
更新于2024-07-22
收藏 3.47MB PDF 举报
无线通信是现代通信领域的重要组成部分,它的发展历程与技术革新深深地影响着人们的生活和工作方式。在Andrea Goldsmith教授的斯坦福大学无线通信标准教材中,读者可以系统地了解到无线通信的历史、愿景、面临的挑战和现有的广泛应用。
首先,章节1概述了无线通信的全貌。历史部分追溯了无线通信的起源,从早期的无线电通信到现代移动通信系统的演变,包括早期的蜂窝电话网络(如1G、2G等)的兴起。作者强调了无线通信如何从最初的好奇和探索,逐渐发展成为现代社会不可或缺的基础设施。
接下来,无线通信的愿景被讨论,探讨了未来可能的技术趋势,如5G、6G网络的超高速度、低延迟和大规模连接能力。这些技术将推动物联网、自动驾驶和远程医疗等领域的进步。
技术问题章节着重于无线信号传输中的关键要素,如路径损耗、多径效应、阴影衰落等,这些都是影响通信质量的重要因素。此外,还提到了多种无线系统的技术特性,如:
- 移动电话系统:包括不同代际的蜂窝网络,如GSM、CDMA、LTE和5G,它们各自的技术特点、频谱利用率和数据传输效率。
- 脱线电话:传统的无绳电话技术,如DECT,以及现代的Wi-Fi通话,展示了无线通信在个人通信中的多样性。
- 无线局域网(WLAN):Wi-Fi技术的兴起及其在家庭、办公室和公共热点的应用。
- 宽带无线接入:WiMAX、Wi-Fi Direct和Wi-Fi 6等技术,为用户提供更宽广的数据传输通道。
- 无线寻呼系统:曾经流行的通信方式,在移动通信普及后逐渐被淘汰,但仍有一定的应用领域。
- 卫星网络:卫星通信在远程覆盖和国际通信中的重要作用,以及全球定位系统(GPS)的普及。
- 蓝牙和Zigbee:这两种低功耗无线技术,广泛应用于物联网设备的短距离连接。
- 超宽带(UWB)技术:提供极高数据传输速率的短距离通信,用于室内定位和高速数据传输。
第1.5节深入探讨了无线频谱的管理,介绍了如何通过频谱分配协议来确保高效利用资源。这涉及到频谱划分、频谱共享策略以及针对现有系统的具体分配情况。
最后,标准制定对于无线通信至关重要,章节1.6涵盖了各种国际和行业标准,如IEEE、3GPP和ITU-R等,它们定义了技术规范和接口,促进了技术的统一和互操作性。
无线通信不仅仅是技术的堆砌,它涉及的是人类对信息传递的深刻理解和对未来的前瞻性思考。随着科技的不断演进,无线通信将继续引领通信行业的变革,塑造我们生活的方方面面。
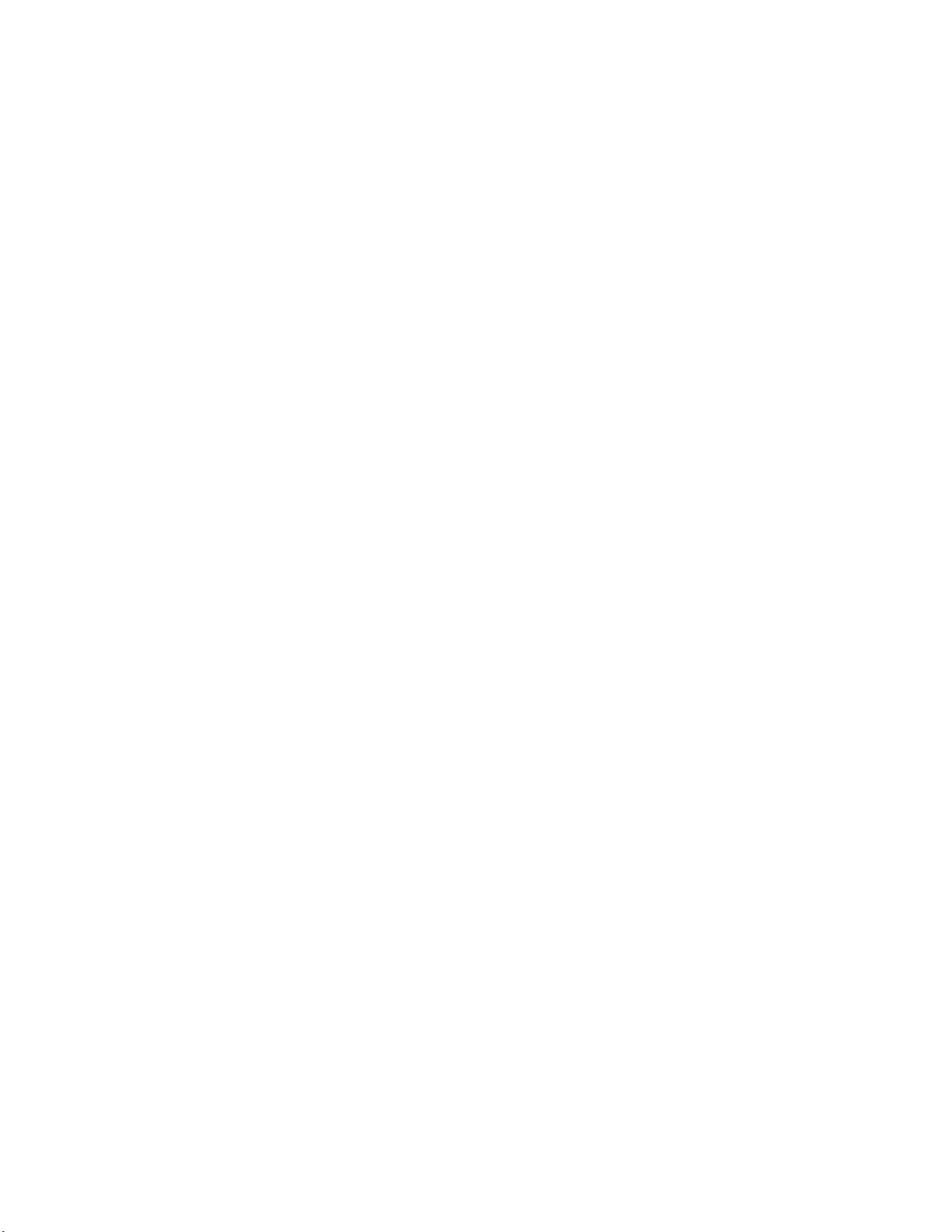
different applications make it difficult to build one wireless system that can efficiently satisfy all these requirements
simultaneously. Wired networks typically integrate the diverse requirements of different using a single protocol.
This integration requires that the most stringent requirements for all applications be met simultaneously. While this
may be possible on some wired networks, with data rates on the order of Gbps and BERs on the order of 10
−12
,
it is not possible on wireless networks, which have much lower data rates and higher BERs. For these reasons, at
least in the near future, wireless systems will continue to be fragmented, with different protocols tailored to support
the requirements of different applications.
The exponential growth of cellular telephone use and wireless Internet access have led to great optimism about
wireless technology in general. Obviously not all wireless applications will flourish. While many wireless systems
and companies have enjoyed spectacular success, there have also been many failures along the way, including
first generation wireless LANs, the Iridium satellite system, wide area data services such as Metricom, and fixed
wireless access (wireless “cable”) to the home. Indeed, it is impossible to predict what wireless failures and
triumphs lie on the horizon. Moreover, there must be sufficient flexibility and creativity among both engineers and
regulators to allow for accidental successes. It is clear, however, that the current and emerging wireless systems of
today coupled with the vision of applications that wireless can enable insure a bright future for wireless technology.
1.3 Technical Issues
Many technical challenges must be addressed to enable the wireless applications of the future. These challenges
extend across all aspects of the system design. As wireless terminals add more features, these small devices must
incorporate multiple modes of operation to support the different applications and media. Computers process voice,
image, text, and video data, but breakthroughs in circuit design are required to implement the same multimode
operation in a cheap, lightweight, handheld device. Since consumers don’t want large batteries that frequently
need recharging, transmission and signal processing in the portable terminal must consume minimal power. The
signal processing required to support multimedia applications and networking functions can be power-intensive.
Thus, wireless infrastructure-based networks, such as wireless LANs and cellular systems, place as much of the
processing burden as possible on fixed sites with large power resources. The associated bottlenecks and single
points-of-failure are clearly undesirable for the overall system. Ad hoc wireless networks without infrastructure
are highly appealing for many applications due to their flexibility and robustness. For these networks all processing
and control must be performed by the network nodes in a distributed fashion, making energy-efficiency challenging
to achieve. Energy is a particularly critical resource in networks where nodes cannot recharge their batteries, for
example in sensing applications. Network design to meet the application requirements under such hard energy
constraints remains a big technological hurdle. The finite bandwidth and random variations of wireless channels
also requires robust applications that degrade gracefully as network performance degrades.
Design of wireless networks differs fundamentally from wired network design due to the nature of the wireless
channel. This channel is an unpredictable and difficult communications medium. First of all, the radio spectrum
is a scarce resource that must be allocated to many different applications and systems. For this reason spectrum
is controlled by regulatory bodies both regionally and globally. A regional or global system operating in a given
frequency band must obey the restrictions for that band set forth by the corresponding regulatory body. Spectrum
can also be very expensive since in many countries spectral licenses are often auctioned to the highest bidder.
In the U.S. companies spent over nine billion dollars for second generation cellular licenses, and the auctions in
Europe for third generation cellular spectrum garnered around 100 billion dollars. The spectrum obtained through
these auctions must be used extremely efficiently to get a reasonable return on its investment, and it must also
be reused over and over in the same geographical area, thus requiring cellular system designs with high capacity
and good performance. At frequencies around several Gigahertz wireless radio components with reasonable size,
power consumption, and cost are available. However, the spectrum in this frequency range is extremely crowded.
5
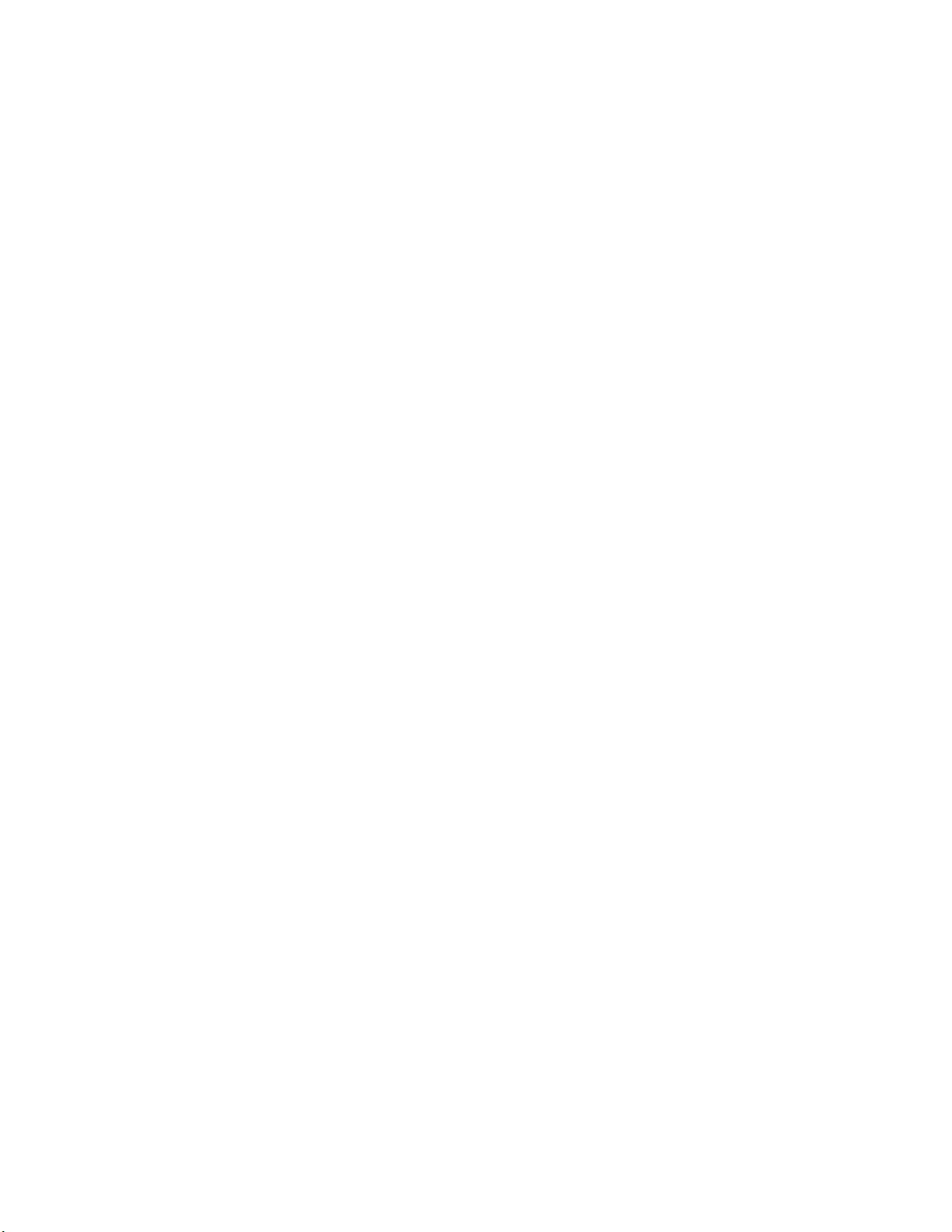
Thus, technological breakthroughs to enable higher frequency systems with the same cost and performance would
greatly reduce the spectrum shortage. However, path loss at these higher frequencies is larger, thereby limiting
range, unless directional antennas are used.
As a signal propagates through a wireless channel, it experiences random fluctuations in time if the transmitter,
receiver, or surrounding objects are moving, due to changing reflections and attenuation. Thus, the characteristics
of the channel appear to change randomly with time, which makes it difficult to design reliable systems with
guaranteed performance. Security is also more difficult to implement in wireless systems, since the airwaves are
susceptible to snooping from anyone with an RF antenna. The analog cellular systems have no security, and one
can easily listen in on conversations by scanning the analog cellular frequency band. All digital cellular systems
implement some level of encryption. However, with enough knowledge, time and determination most of these
encryption methods can be cracked and, indeed, several have been compromised. To support applications like
electronic commerce and credit card transactions, the wireless network must be secure against such listeners.
Wireless networking is also a significant challenge. The network must be able to locate a given user wherever
it is among billions of globally-distributed mobile terminals. It must then route a call to that user as it moves at
speeds of up to 100 Km/hr. The finite resources of the network must be allocated in a fair and efficient manner
relative to changing user demands and locations. Moreover, there currently exists a tremendous infrastructure of
wired networks: the telephone system, the Internet, and fiber optic cable, which should be used to connect wireless
systems together into a global network. However, wireless systems with mobile users will never be able to compete
with wired systems in terms of data rates and reliability. Interfacing between wireless and wired networks with
vastly different performance capabilities is a difficult problem.
Perhaps the most significant technical challenge in wireless network design is an overhaul of the design
process itself. Wired networks are mostly designed according to a layered approach, whereby protocols associated
with different layers of the system operation are designed in isolation, with baseline mechanisms to interface
between layers. The layers in a wireless systems include the link or physical layer, which handles bit transmissions
over the communications medium, the access layer, which handles shared access to the communications medium,
the network and transport layers, which routes data across the network and insure end-to-end connectivity and data
delivery, and the application layer, which dictates the end-to-end data rates and delay constraints associated with
the application. While a layering methodology reduces complexity and facilitates modularity and standardization,
it also leads to inefficiency and performance loss due to the lack of a global design optimization. The large
capacity and good reliability of wired networks make these inefficiencies relatively benign for many wired network
applications, although it does preclude good performance of delay-constrained applications such as voice and
video. The situation is very different in a wireless network. Wireless links can exhibit very poor performance,
and this performance along with user connectivity and network topology changes over time. In fact, the very
notion of a wireless link is somewhat fuzzy due to the nature of radio propagation and broadcasting. The dynamic
nature and poor performance of the underlying wireless communication channel indicates that high-performance
networks must be optimized for this channel and must be robust and adaptive to its variations, as well as to network
dynamics. Thus, these networks require integrated and adaptive protocols at all layers, from the link layer to the
application layer. This cross-layer protocol design requires interdiciplinary expertise in communications, signal
processing, and network theory and design.
In the next section we give an overview of the wireless systems in operation today. It will be clear from
this overview that the wireless vision remains a distant goal, with many technical challenges to overcome. These
challenges will be examined in detail throughout the book.
6
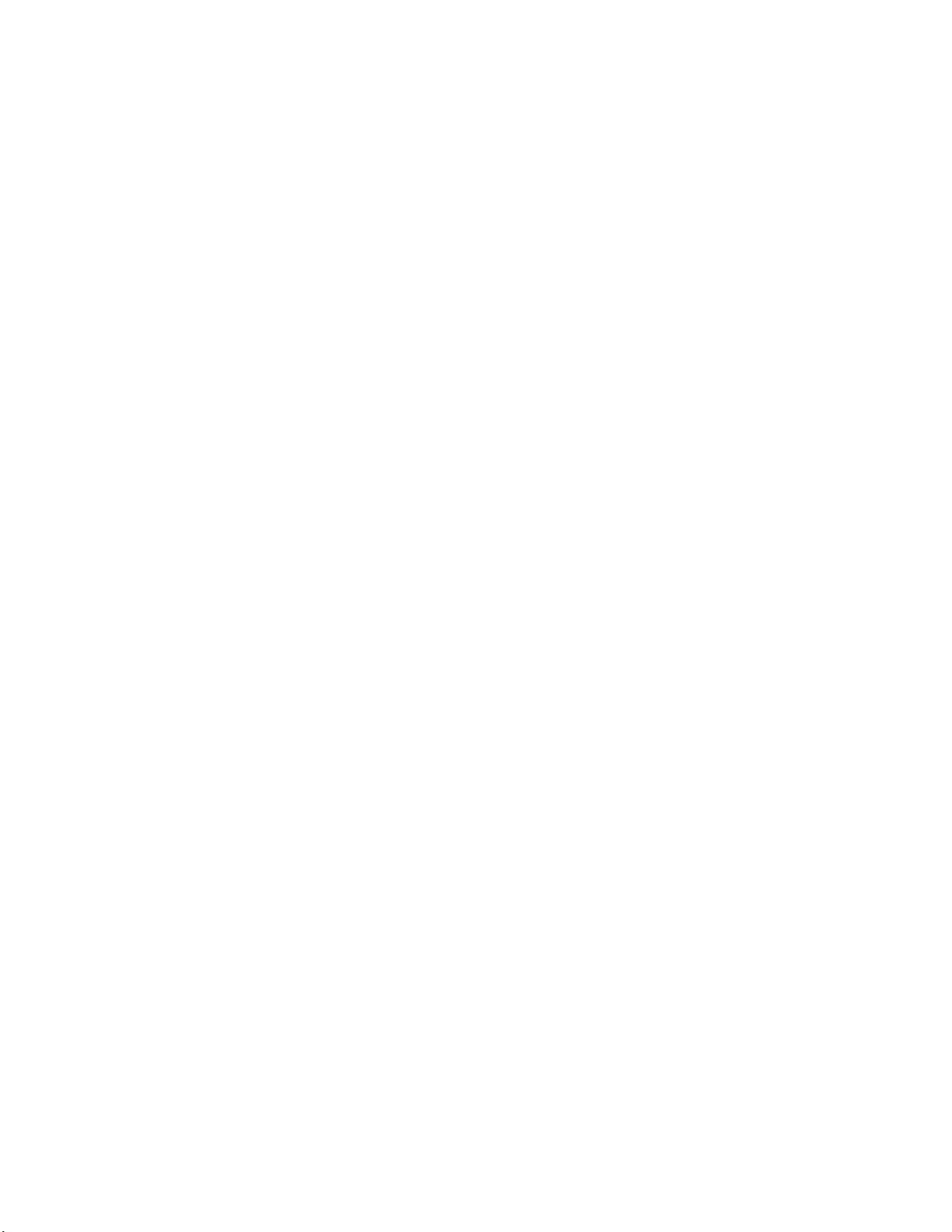
1.4 Current Wireless Systems
This section provides a brief overview of current wireless systems in operation today. The design details of these
system are constantly evolving, with new systems emerging and old ones going by the wayside. Thus, we will
focus mainly on the high-level design aspects of the most common systems. More details on wireless system
standards can be found in [1, 2, 3] A summary of the main wireless system standards is given in Appendix D.
1.4.1 Cellular Telephone Systems
Cellular telephone systems are extremely popular and lucrative worldwide: these are the systems that ignited the
wireless revolution. Cellular systems provide two-way voice and data communication with regional, national, or
international coverage. Cellular systems were initially designed for mobile terminals inside vehicles with antennas
mounted on the vehicle roof. Today these systems have evolved to support lightweight handheld mobile terminals
operating inside and outside buildings at both pedestrian and vehicle speeds.
The basic premise behind cellular system design is frequency reuse, which exploits the fact that signal power
falls off with distance to reuse the same frequency spectrum at spatially-separated locations. Specifically, the
coverage area of a cellular system is divided into nonoverlapping cells where some set of channels is assigned
to each cell. This same channel set is used in another cell some distance away, as shown in Figure 1.1, where
C
i
denotes the channel set used in a particular cell. Operation within a cell is controlled by a centralized base
station, as described in more detail below. The interference caused by users in different cells operating on the same
channel set is called intercell interference. The spatial separation of cells that reuse the same channel set, the reuse
distance, should be as small as possible so that frequencies are reused as often as possible, thereby maximizing
spectral efficiency. However, as the reuse distance decreases, intercell interference increases, due to the smaller
propagation distance between interfering cells. Since intercell interference must remain below a given threshold
for acceptable system performance, reuse distance cannot be reduced below some minimum value. In practice it
is quite difficult to determine this minimum value since both the transmitting and interfering signals experience
random power variations due to the characteristics of wireless signal propagation. In order to determine the best
reuse distance and base station placement, an accurate characterization of signal propagation within the cells is
needed.
Initial cellular system designs were mainly driven by the high cost of base stations, approximately one million
dollars apiece. For this reason early cellular systems used a relatively small number of cells to cover an entire city
or region. The cell base stations were placed on tall buildings or mountains and transmitted at very high power with
cell coverage areas of several square miles. These large cells are called macrocells. Signal power was radiated
uniformly in all directions, so a mobile moving in a circle around the base station would have approximately
constant received power if the signal was not blocked by an attenuating object. This circular contour of constant
power yields a hexagonal cell shape for the system, since a hexagon is the closest shape to a circle that can cover a
given area with multiple nonoverlapping cells.
Cellular systems in urban areas now mostly use smaller cells with base stations close to street level transmit-
ting at much lower power. These smaller cells are called microcells or picocells, depending on their size. This
evolution to smaller cells occured for two reasons: the need for higher capacity in areas with high user density and
the reduced size and cost of base station electronics. A cell of any size can support roughly the same number of
users if the system is scaled accordingly. Thus, for a given coverage area a system with many microcells has a
higher number of users per unit area than a system with just a few macrocells. In addition, less power is required
at the mobile terminals in microcellular systems, since the terminals are closer to the base stations. However, the
evolution to smaller cells has complicated network design. Mobiles traverse a small cell more quickly than a large
cell, and therefore handoffs must be processed more quickly. In addition, location management becomes more
complicated, since there are more cells within a given area where a mobile may be located. It is also harder to
7
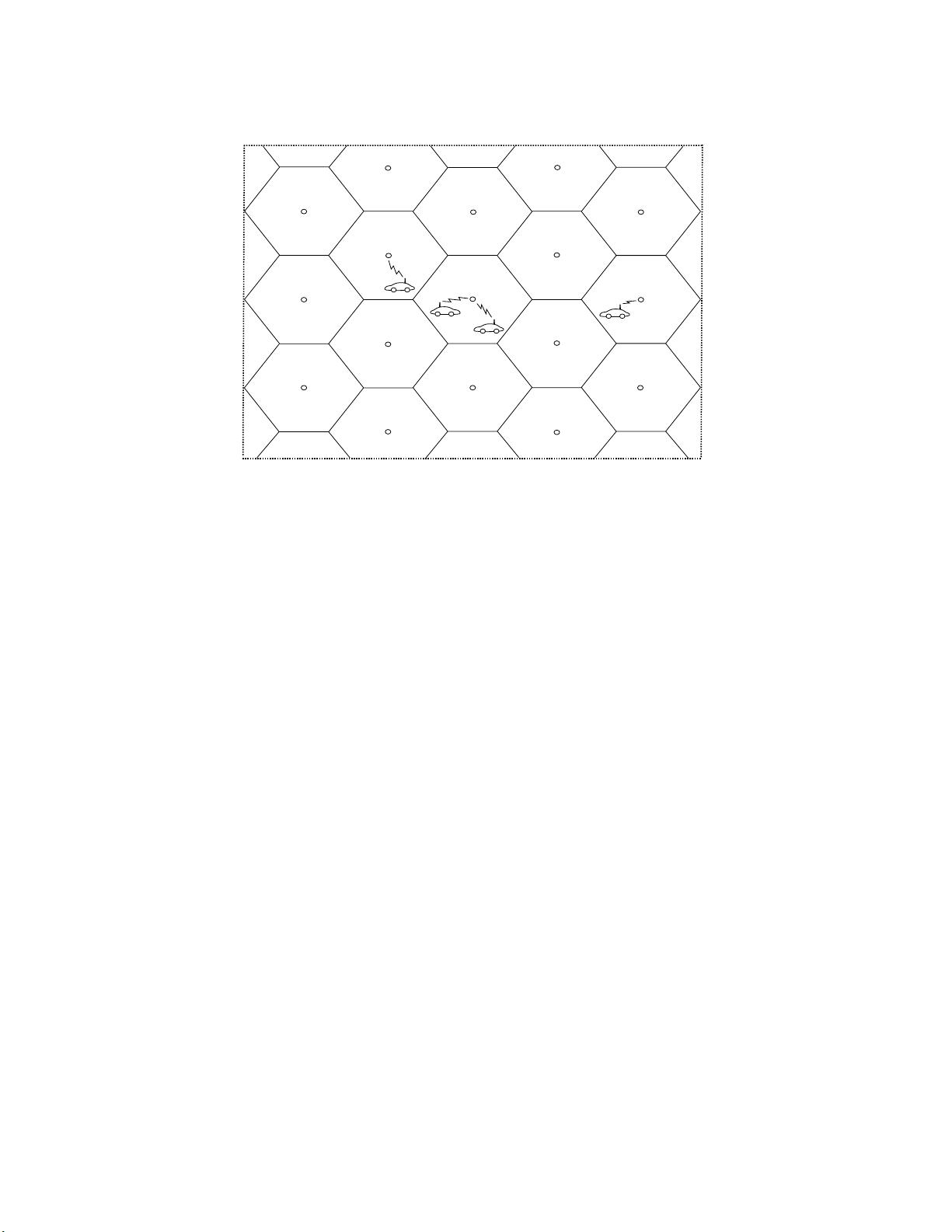
3
3
3
2
1
3
1
2
3
2
1
1
1
2
Base
Station
1
1
2
C
C
C
C
C
C
C
C
C
C
C
C
C
C
C
C
C
Figure 1.1: Cellular Systems.
develop general propagation models for small cells, since signal propagation in these cells is highly dependent
on base station placement and the geometry of the surrounding reflectors. In particular, a hexagonal cell shape is
generally not a good approximation to signal propagation in microcells. Microcellular systems are often designed
using square or triangular cell shapes, but these shapes have a large margin of error in their approximation to
microcell signal propagation [9].
All base stations in a given geographical area are connected via a high-speed communications link to a mobile
telephone switching office (MTSO), as shown in Figure 1.2. The MTSO acts as a central controller for the network,
allocating channels within each cell, coordinating handoffs between cells when a mobile traverses a cell boundary,
and routing calls to and from mobile users. The MTSO can route voice calls through the public switched telephone
network (PSTN) or provide Internet access. A new user located in a given cell requests a channel by sending a call
request to the cell’s base station over a separate control channel. The request is relayed to the MTSO, which accepts
the call request if a channel is available in that cell. If no channels are available then the call request is rejected. A
call handoff is initiated when the base station or the mobile in a given cell detects that the received signal power for
that call is approaching a given minimum threshold. In this case the base station informs the MTSO that the mobile
requires a handoff, and the MTSO then queries surrounding base stations to determine if one of these stations can
detect that mobile’s signal. If so then the MTSO coordinates a handoff between the original base station and the
new base station. If no channels are available in the cell with the new base station then the handoff fails and the
call is terminated. A call will also be dropped if the signal strength between a mobile and its base station drops
below the minimum threshold needed for communication due to random signal variations.
The first generation of cellular systems used analog communications, since they were primarily designed in
the 1960’s, before digital communications became prevalent. Second generation systems moved from analog to
digital due to its many advantages. The components are cheaper, faster, smaller, and require less power. Voice
quality is improved due to error correction coding. Digital systems also have higher capacity than analog systems
since they can use more spectrally-efficient digital modulation and more efficient techniques to share the cellular
spectrum. They can also take advantage of advanced compression techniques and voice activity factors. In addition,
8
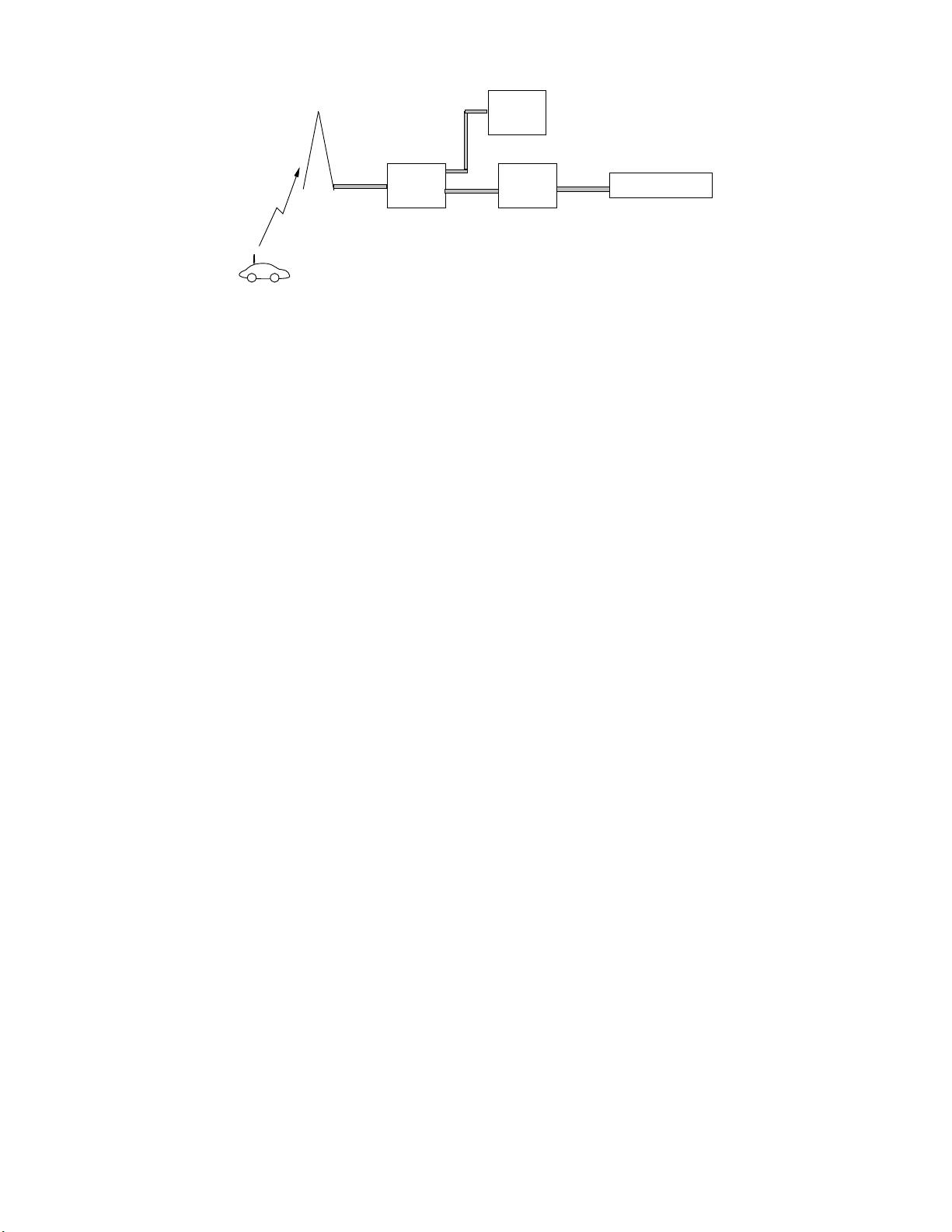
MOBILE
TELEPHONE
SWITCHING
OFFICE
LOCAL
EXCHANGE
LONG−DISTANCE
NETWORK
PHONE
CELLULAR
BASE
STATION
INTERNET
Figure 1.2: Current Cellular Network Architecture
encryption techniques can be used to secure digital signals against eavesdropping. Digital systems can also offer
data services in addition to voice, including short messaging, email, Internet access, and imaging capabilities
(camera phones). Due to their lower cost and higher efficiency, service providers used aggressive pricing tactics to
encourage user migration from analog to digital systems, and today analog systems are primarily used in areas with
no digital service. However, digital systems do not always work as well as the analog ones. Users can experience
poor voice quality, frequent call dropping, and spotty coverage in certain areas. System performance has certainly
improved as the technology and networks mature. In some areas cellular phones provide almost the same quality as
landline service. Indeed, some people have replaced their wireline telephone service inside the home with cellular
service.
Spectral sharing in communication systems, also called multiple access, is done by dividing the signaling
dimensions along the time, frequency, and/or code space axes. In frequency-division multiple access (FDMA) the
total system bandwidth is divided into orthogonal frequency channels. In time-division multiple access (TDMA)
time is divided orthogonally and each channel occupies the entire frequency band over its assigned timeslot. TDMA
is more difficult to implement than FDMA since the users must be time-synchronized. However, it is easier to ac-
commodate multiple data rates with TDMA since multiple timeslots can be assigned to a given user. Code-division
multiple access (CDMA) is typically implemented using direct-sequence or frequency-hopping spread spectrum
with either orthogonal or non-orthogonal codes. In direct-sequence each user modulates its data sequence by a
different chip sequence which is much faster than the data sequence. In the frequency domain, the narrowband
data signal is convolved with the wideband chip signal, resulting in a signal with a much wider bandwidth than
the original data signal. In frequency-hopping the carrier frequency used to modulate the narrowband data signal
is varied by a chip sequence which may be faster or slower than the data sequence. This results in a modulated
signal that hops over different carrier frequencies. Typically spread spectrum signals are superimposed onto each
other within the same signal bandwidth. A spread spectrum receiver separates out each of the distinct signals by
separately decoding each spreading sequence. However, for non-orthogonal codes users within a cell interfere
with each other (intracell interference) and codes that are reused in other cells cause intercell interference. Both
the intracell and intercell interference power is reduced by the spreading gain of the code. Moreover, interference
in spread spectrum systems can be further reduced through multiuser detection and interference cancellation. More
details on these different techniques for spectrum sharing and their performance analysis will be given in Chap-
ters 13-14. The design tradeoffs associated with spectrum sharing are very complex, and the decision of which
technique is best for a given system and operating environment is never straightforward.
Efficient cellular system designs are interference-limited, i.e. the interference dominates the noise floor since
otherwise more users could be added to the system. As a result, any technique to reduce interference in cellular
systems leads directly to an increase in system capacity and performance. Some methods for interference reduction
in use today or proposed for future systems include cell sectorization, directional and smart antennas, multiuser
9
剩余571页未读,继续阅读
2008-09-09 上传
2010-03-16 上传
2009-12-13 上传
2010-05-25 上传
2009-02-05 上传
2011-07-13 上传


songzhe6775
- 粉丝: 0
- 资源: 5
上传资源 快速赚钱
我的内容管理 展开
我的资源 快来上传第一个资源
我的收益
登录查看自己的收益我的积分 登录查看自己的积分
我的C币 登录后查看C币余额
我的收藏
我的下载
下载帮助

最新资源
- CSharp算法Cambridge University Press - Data Structures and Algorithms Using C# (Mar 2007)
- 华为_Verilog HDL入门教程
- 基于CAN总线的β-甘露聚糖酶发酵控制系统的研究
- 2009年考研计算机专业基础综合大纲
- altera nios从入门到精通
- 类人机器人手臂控制系统设计
- 单元测试测试用例设计
- Windows文件系统过滤驱动开发教程(第二版)
- 常用485芯片通信协议
- 232-485转接电路
- linux多线程编程手册.pdf
- Tornado使用指南
- x5045简介资料 设计的好帮手
- 《MiniGUI 用户手册》.pdf
- cc2500中文数据手册
- hibernate in action(第二版)
安全验证
文档复制为VIP权益,开通VIP直接复制
