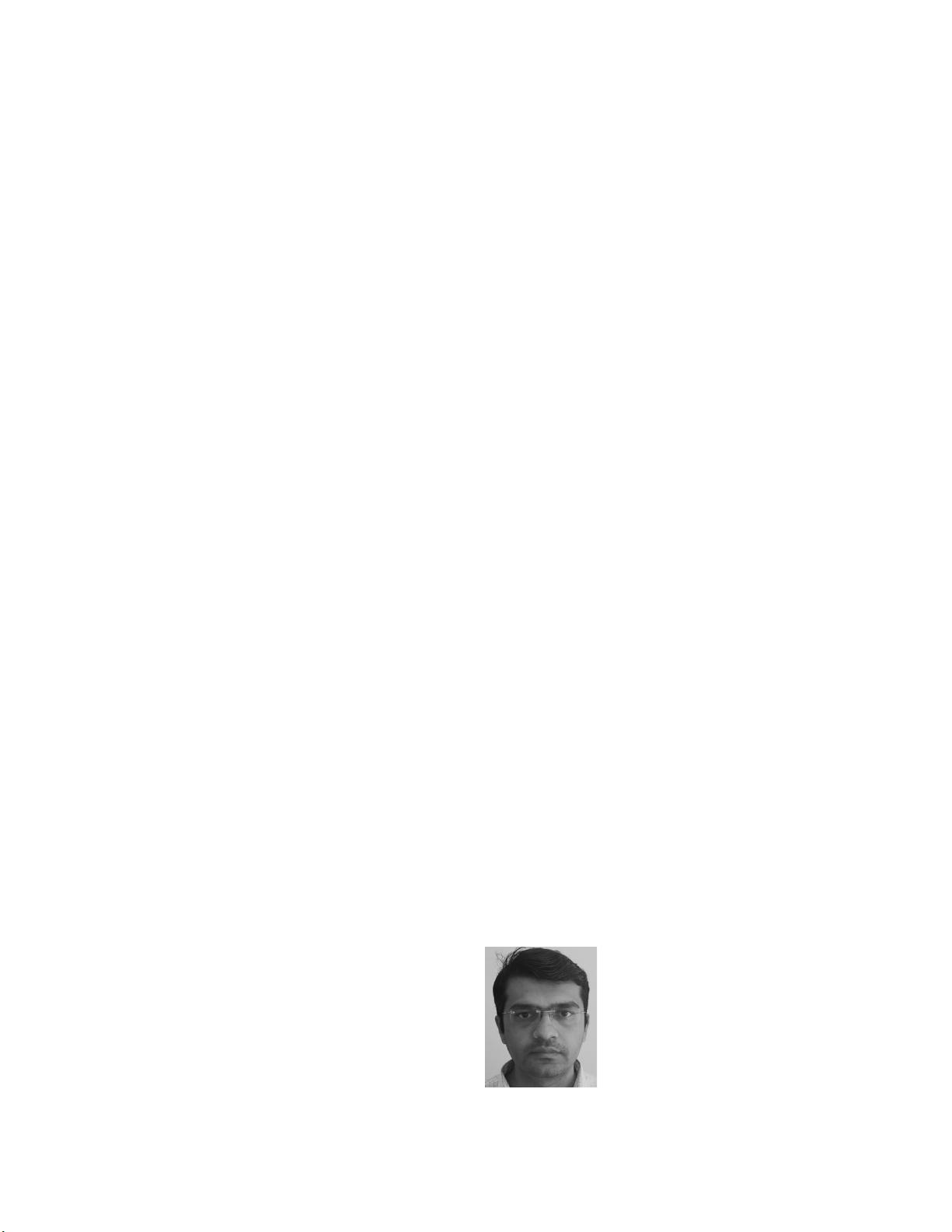
MEHTA et al. : ON-CHIP RELAXATION OSCILLATOR IN 5-nm FinFET 2907
Table I summarizes the performance of the proposed oscil-
lator a nd compares it with previously publishe d state-of-the-art
works. The proposed design operates at 7 7 MHz which is one
of the h ighest among the recently published works. Thanks
to the small feature size of the 5-nm FinFET process, the
proposed oscillator achieves the lowest area even though it
uses a bandgap reference for generating the reference voltage
and reference current sources. Compared with [4], [5], the TIE
jitter is reduced by 3×. While the TIE is comparable to [15],
the thermal stability is 6× better. The frequency variations
with supply and temperature reported in Table I for this design
are the worst case values from 16 and eight chip samples,
respectively.
V. C
ONCLUSION
A relaxation oscillator using a FEF loop is describ ed for
securing SoCs against physical clock attacks. From a security
perspective, the
ON-chip oscillator should achieve good sta-
bility with supply and temperature as well as good TIE jitter
and period jitter. The oscillator using an FEF loop is shown to
meet these requirements using circuit techniques such as CDS-
based error-amplifier, current splitter, and reuse of loop-filter
as a hold capacitor. Realized in 5-nm FinFET, the 77-MHz
oscillator achieves 11-μW/MHz power efficiency and a TIE
of 2.81 ns over 10 K cycles with a period jitter of 60 ps. The
worst case frequency stability over 1 .1–1.35-V supply and the
temperature range of −40
◦
C to 125
◦
Cis±0.25% and ±0.3%,
respectively. This performance combined with the use of no
external components makes the proposed oscillator well-suited
for securing the boot-up process and as a reference for
ON-chip
clock monitors.
A
CKNOWL EDGMENT
The authors thank Brian Zimmer and Sudhir Kudva for
layout help and Neil Pham for equipment support.
R
EFERENCES
[1] C. H. Kim and J.-J. Quisquater, “Faults, injection methods, and fault
attacks,” IEEE Design Test Comput., vol. 24, no. 6, pp. 544–545,
Nov. 2007.
[2] T. I. Wiki. (Jul. 2009). 0x24000 Segment Overflow. Accessed:
Jan. 13, 2022. [Online]. Available: https://www.theiphonewiki.com/
wiki/0x24000_Segment_Overflo w
[3] F. Basse. (Oct. 2016). Amlogic S905 SoC: Bypassing the (not so)
Secure Boot to Dump the BootROM. Accessed: Jan. 13, 2022. [Online].
Av ailable: https://fredericb .info/2016/10/amlogic-s905-soc-bypassing-
not-so.html
[4] Y. Tokunaga, S. Sakiyama, A. Matsumoto, and S. Dosho, “An on-chip
CMOS relaxation oscillator with voltage averaging feedback,” IEEE
J . Solid-State Circuits, vol. 45, no. 6, pp. 1150–1158, Jun. 2010.
[5] Y. Cao, P. Leroux, W. De Cock, and M. Steyaert, “A 63,000 Q-factor
relaxation oscillator with switched-capacitor integrated error feedback,”
in IEEE Int. Solid-State Circuits Conf. (ISSCC) Dig. Tech. Papers,
Feb. 2013, pp. 186–187.
[6] K. Pappu, G. P. Reitsma, and S. Bapat, “5.4 frequency-locked-loop ring
oscillator with 3 ns peak-to-peak accumulated jitter in 1 ms time window
for high-resolution frequency counting,” in IEEE Int. Solid-State Circuits
Conf. (ISSCC) Dig. Tech. Papers, Feb. 2017, pp. 92–93.
[7] T. T okairin et al., “A 280 nW, 100 kHz, 1-cycle start-up time, on-
chip CMOS relaxation oscillator employing a feedforward period control
scheme,” in Proc. Symp. VLSI Circuits (VLSIC), Jun. 2012, pp. 16–17.
[8] H. Jiang, S. Pan, C. Gurleyuk, and K. A. A. Makinwa, “A 0.14 mm
2
16 MHz CMOS RC frequency reference with a 1-point trimmed inac-
curacy of ±400 ppm from −45
◦
Cto85
◦
C,” in IEEE Int. Solid-State
Circuits Conf. (ISSCC) Dig. Tech. Papers, Feb. 2021, pp. 436–438.
[9] K. Lasanen, E. Raisanen-Ruotsalainen, and J. Kostamovaara, “A 1-V,
self adjusting, 5-MHz CMOS RC-oscillator ,” in Proc. IEEE Int. Symp.
Circuits Syst., vol. 4, May 2002, p. 4.
[10] N. Mehta, S. Tell, W. Turner, L. Tatro, G. Goh, and C. T. Gray,
“A 77 MHz relaxation oscillator in 5nm FinFET with 3 ns TIE ov e r
10 K cycles and ±0.3% thermal stability using frequency-error feed-
back loop,” in Proc. IEEE Asian Solid-State Circuits Conf. (A-SSCC),
Nov. 2021, pp. 1–3.
[11] U. Denier, “Analysis and design of an ultralow-power CMOS relaxation
oscillator ,” IEEE Trans. Circuits Syst. I, Reg . Papers, vol. 57, no. 8,
pp. 1973–1982, Aug. 2010.
[12] C. C. Enz and G. C. Temes, “Circuit techniques for reducing the effects
of op-amp imperfections: Autozeroing, correlated double sampling, and
chopper stabilization,” Proc. IEEE, vol. 84, no. 11, pp. 1584–1614,
Nov. 1996.
[13] D. Griffith, P. T. Røine, J. Murdock, and R. Smith, “A 190 nW 33 kHz
RC oscillator with ±0.21% temperature stability and 4 ppm long-term
stability,” in IEEE Int. Solid-State Circuits Conf. (ISSCC) Dig. Tech.
Papers, Feb. 2014, pp. 300–301.
[14] J. Jung, I.-H. Kim, S.-J. Kim, Y. Lee, and J.-H. Chun, “A 1.08-nW/kHz
13.2-ppm/
◦
C self-biased timer using temperature-insensitive resistive
current,” IEEE J. Solid-State Circuits, vol. 53, no. 8, pp. 2311–2318,
Aug. 2018.
[15] J. Lee, A. K. George, and M. Je, “An ultra-low-noise swing-boosted
differential relaxation oscillator in 0.18-μmCMOS,”IEEE J. Solid-State
Circuits, vol. 55, no. 9, pp. 2489–2497, Sep. 2020.
[16] K.-S. Park et al., “A second-order temperature compensated 1μW/MHz
100 MHz RC oscillator with ±140 ppm inaccuracy from −40
◦
Cto
95
◦
C,” in Proc. IEEE Custom Integr. Circuits Conf. (CICC), Apr. 2021,
pp. 1–2.
[17] K.-M. Lei, P.-I. Mak, and R. P. Martins, “A 0.35-V 5,200-μm
2
2.1-MHz
temperature-resilient relaxation oscillator with 667 fJ/cycle energy effi-
ciency using an asymmetric swing-boosted RC network and a dual-path
comparator,” IEEE J. Solid-State Circuits, vol. 56, no. 9, pp. 2701–2710,
Sep. 2021.
[18] J. Lee, P. Park, S. Cho, and M. Je, “A 4.7 MHz 53 μW fully differential
CMOS reference clock oscillator with −22 dB worst-case PSNR for
miniaturized SoCs,” in IEEE Int. Solid-State Circuits Conf. (ISSCC) Dig.
Tech. Papers, Feb. 2015, pp. 1–3.
[19] J. Koo, K.-S. Moon, B. Kim, H.-J. Park, and J.-Y. Sim,
“5.5 A quadrature relaxation oscillator with a process-induced
frequency-error compensation loop,” in IEEE Int. Solid-State Circuits
Conf. (ISSCC) Dig. Tech. Papers, Feb. 2017, pp. 94–95.
[20] A. L. S. Loke et al., “Analog/mixed-signal design challenges in
7-nm CMOS and beyond,” in Proc. IEEE Custom Integr. Circuits Conf.
(CICC), Apr. 2018, pp. 1–8.
Nandish Mehta (Senior Member, IEEE) received
the M.Sc. degree in microelectronics from the Delft
Uni versity of T echnology, Delft, The Netherlands,
in 2013, and the Ph.D. degree in electrical engi-
neering and computer science from the University of
California at Berkeley, Berkeley, CA, USA, in 2019.
He held internships with Apple Inc., Cupertino,
CA, USA; Ayar labs, Emeryville, CA, USA; and
Broadcom Inc., Bunnik, The Netherlands. He is
currently with Nvidia Corporation, Santa Clara, CA,
USA. His research focuses on applications of inte-
grated silicon photonics, analog-mixed signal circuits, and serial links.
Dr. Mehta was a recipient of the Foundation for Excellence Scholarship
in 2005, the Intel Scholarship in 2008, the NXP Scholarship in 2011, the
Department of EECS Fellowship in 2014, the ADI Outstanding Student
Designer Award in 2018, and the IEEE SSCS Predoctoral Award in 2019.