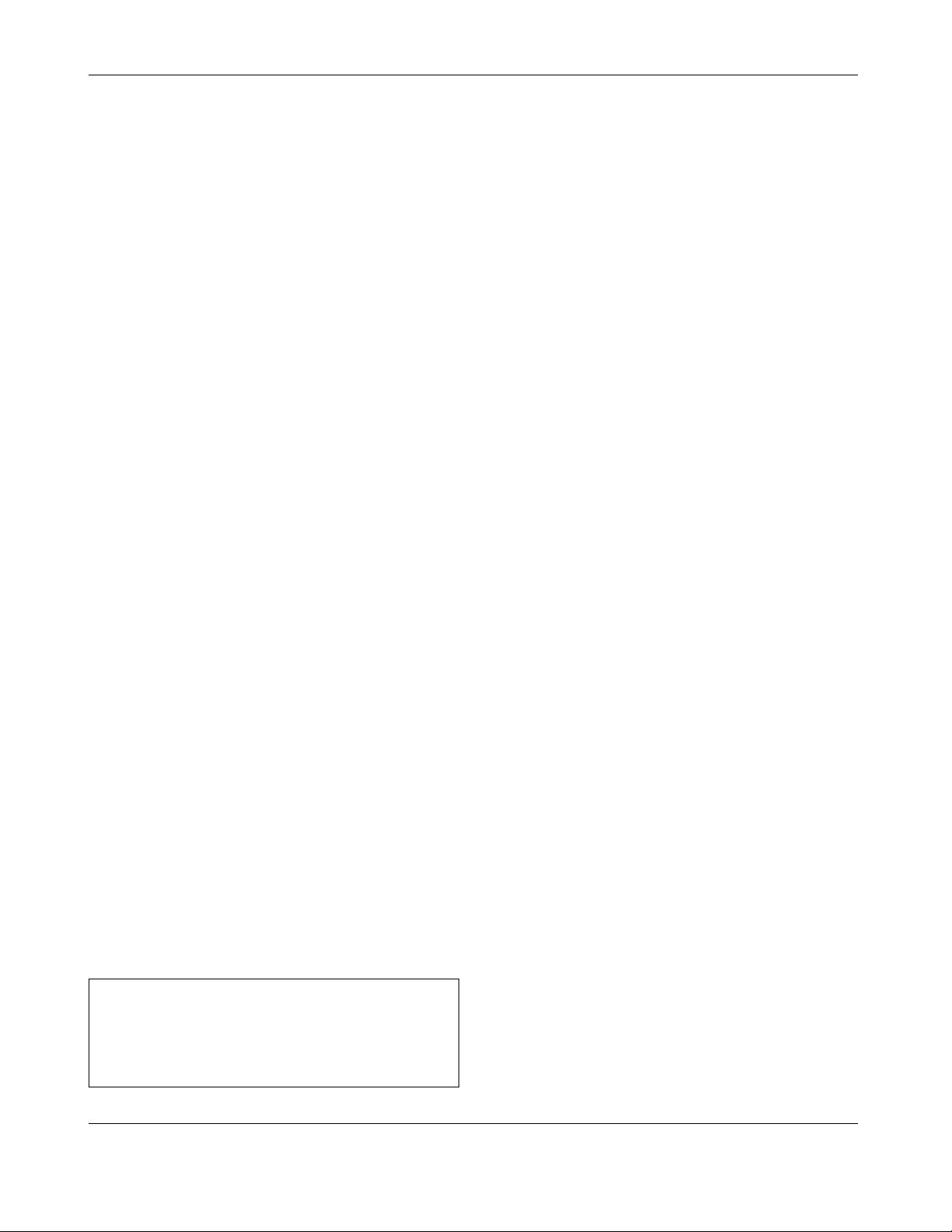
Glial strategy for metabolic
shuttling and neuronal function
Joachim W. Deitmer
Summary
Glial cells serve a variety of functions in nervous
systems, some of which are activated by neurotrans-
mitters released from neurons. Glial cells respond to
these neurotransmitters via receptors, but also take up
some of the transmitters to help terminate the synaptic
process. Among these, glutamate uptake by glial cells is
pivotal to avoid transmitter-mediated excitotoxicity.
Here, a new model is proposed in which glutamate
uptake via the excitatory amino acid transporter (EAAT)
is functionally coupled to other glial transporters, in
particular the sodium-bicarbonate cotransporter (NBC)
and the monocarboxylate transporter (MCT), as well as
other glial functions, such as calcium signalling, a high
potassium conductance and CO
2
consumption. The
central issue of this hypothesis is that the shuttling of
sodium ions and acid/base equivalents, which drive the
metabolite transport across the glial membrane, co-
operate with each other, and hence save energy. As a
result, glutamate removal from synaptic domains and
lactate secretion serving the energy supply to neurons
would be facilitated and could operate with greater
capacity. BioEssays 22:747±752, 2000.
ß 2000 John Wiley & Sons, Inc.
Introduction
Glutamate is the most abundant and versatile excitatory
neurotransmitter in vertebrate, and presumably in all,
nervous systems. It activates a number of ionotropic and
metabotropic receptors in the postsynaptic neuronal mem-
brane, with the precise excitatory response depending on the
kind of receptor activated and its subunit assembly.
(1)
Furthermore, it has become evident in recent years that
glutamate acts not only on neurons but also on glial cells, in
particular on astrocytes in the central nervous system, which
are equipped with different types of glutamate receptors. In
addition, in both neurons and astrocytes, glutamate is taken
up by different isoforms of excitatory amino acid transporters
(EAAT).
(2,3)
Astrocytes seem to play a dominant role, at least
in some brain areas, in removing glutamate from extra-
cellular synaptic domains,
(4,5)
thereby contributing to the
termination of glutamatergic transmission.
(6,7)
The stoichiometry of the glial glutamate uptake transpor-
ter suggests that with each glutamate taken up, three sodium
ions flow into, while one potassium ion leaves, the cell; the
concomitant intracellular acidosis has been explained by the
accompanying flux of one acid equivalent into, or one base
equivalent out of, the cell.
(8,9)
Thus, the transporter is elec-
trogenic, moving one or more net positive charges into the
cell, and, when activated, depolarizes the cell membrane.
Accordingly, glutamate uptake is enhanced by membrane
hyperpolarization, and attenuated by membrane depolariza-
tion, due to the increase or decrease of the potential
gradient, respectively, driving the transport.
The energy demand of neurons and glial cells is believed
to be met mainly by glucose uptake from blood capillaries by
astrocytic endfeet; most of the glucose in the astrocytes is
converted into lactate.
(10,11)
Lactic acid is a major end
product of glucose metabolism in many vertebrate cells and
the production of lactic acid is the main source of intracellular
acidification, especially during anoxia, when oxidative gen-
eration of ATP is suppressed. In the mammalian brain,
lactate is the principal energy source, and is transferred from
astrocytes to neurons by monocarboxylate/proton cotran-
sporters.
(12± 14)
This energy supply is needed by active
neurons, when they fire action potentials and release
neurotransmitters. It has been shown that glucose uptake
and utilization by glial cells, and the energy transfer between
glial cells and neurons, is enhanced by glutamate.
(15)
A large portion of the energy needed by neural cells is
used in active transport, most of which depends directly or
indirectly upon a steep sodium gradient across the cell
membranes. Many ion and substrate transporters (e.g. for
calcium, hydrogen, glucose, glutamate and GABA) are driven
by the sodium gradient, which itself is maintained by the
ATP-consuming sodium/potassium pump (Na/K-ATPase).
Blocking the Na/K-ATPase, for instance with ouabain, leads
to an increase of intracellular sodium, and to a decrease of
the sodium gradient across the cell membrane. The latter
effect, in turn, impairs sodium-dependent processes, such as
glutamate uptake.
(15,16)
Therefore, the Na/K-ATPase is prob-
ably the main consumer of primary chemical energy in the
form of ATP in the brain,
(17)
as in most other animal tissues.
In the brain, three metabolic demands are therefore of
prime importance: (1) the maintenance of a steep sodium
gradient across cell membranes by maintaining a low intra-
cellular sodium concentration (in vertebrate cells, it is usually
BioEssays 22:747±752, ß 2000 John Wiley & Sons, Inc. BioEssays 22.8 747
Abteilung fu
È
r Zoologie, FB Biologie, Universita
È
t Kaiserslautern,
Postfach 3049, D-67653 Kaiserslautern, Germany. E-mail:
deitmer@rhrk.uni-kl.de
Funding agency: Deutsche Forschungsgemeinschaft. Grant numbers:
De 231/11-2,13-1 and SFB 530, TP B1.
Hypothesis