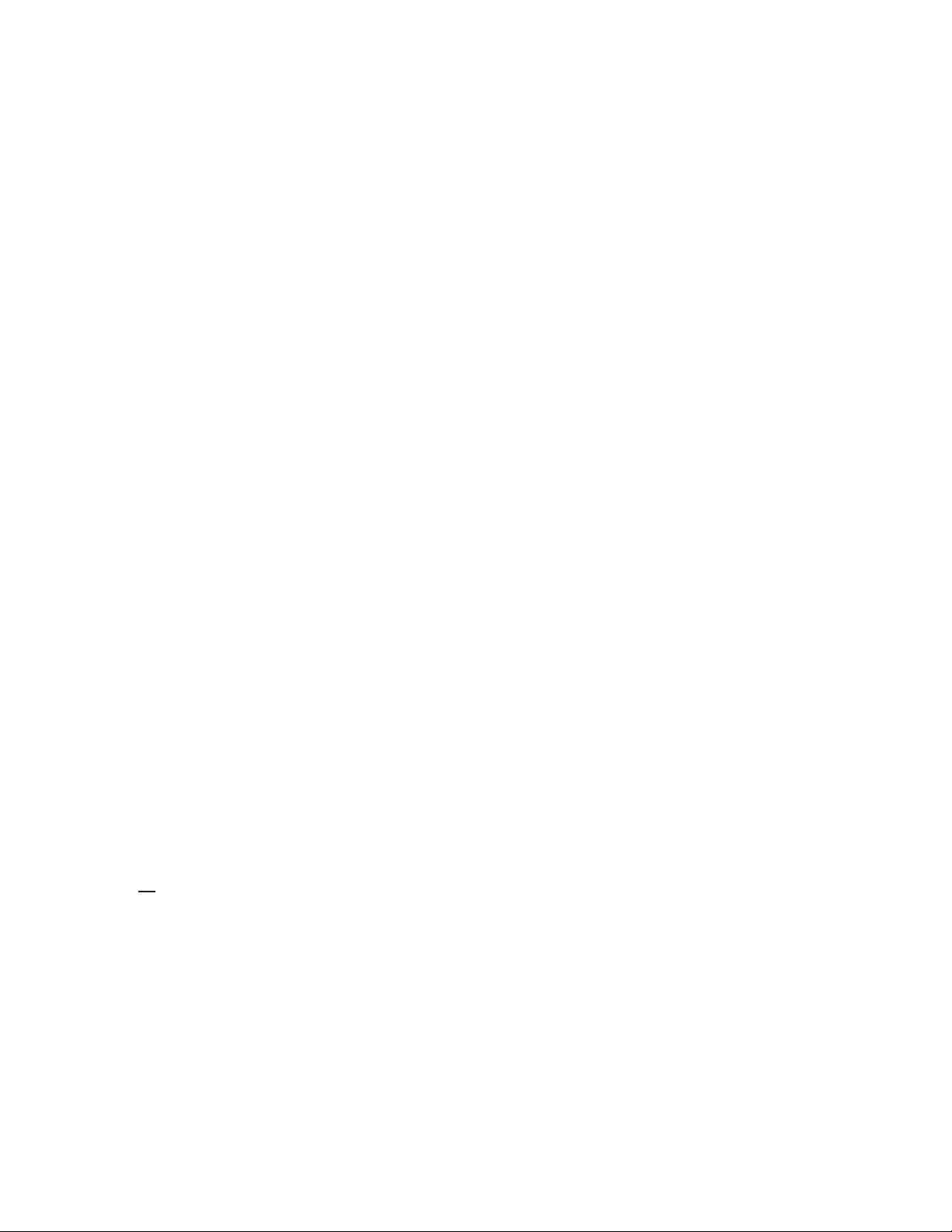
MOUSAVI et al.: 0.4–1.0 GHz, 47 MHop/s FH TXR FRONT END WITH 20 dB IN-BAND BLOCKER REJECTION 1919
the N-path-based correlators. The residual TX signal after the
SIC is orthogonal to the RX LO and, hence, gets reduced
by the correlator. Due to the ultra-fast hopping speed of the
transceiver, the rejection is limited, and a portion of this power
shows up in the receiver channel. The sinc filter response
caused by the fast hopping has its first null at the hop rate
of 47 MHz. Therefore, there is still significant TX energy in
the RX channel. The transmitted signal over one symbol can
be written as shown in (1), where P[t] is the rectangular pulse,
T
h
is the hop time, N is the total number of channels, f
T
i
is the
transmit frequency at the ith hop, and
T
(i) is the necessary
phase at the ith hop at the transmitter to ensure continuity
between the frequency hops
x(t) =
N
i=1
P[t −(i − 1)T
h
]sin[2π f
T
i
t +
T
(i)]. (1)
The signal at the receiver then is given by (2), where f
R
i
is the
receive frequency and
R
(i) is the necessary phase at the ith
hop at the receiver to ensure continuity between the frequency
hops
y(t) =
N
i=1
P[t −(i −1)T
h
]sin[2π f
T
i
t +
T
(i)]e
j2π f
R
i
t+
R
(i)
.
(2)
The spectrum at the receiver can be estimated by perform-
ing the Fourier transform of (2), which can be written as
shown in the equation in the following. Here, we note that
the transmitter and receiver channels are distinct and that the
final spectrum has a sinc shape that is proportional to the
hop-time T
h
. This means that even if RX and TX channels are
different, there is still going to be spill over from TX to RX.
The spacing between TX and RX frequencies alters the phase
and the sinc magnitudes of each of the summation terms that
fall in-band and changes the self-interference that shows up in
the RX band. As the hopping speed increases, T
h
decreases,
which widens the sinc function that causes more energy to
show up in the RX baseband. This problem is normally not
seen at lower hopping speeds, as the sinc main lobe is much
narrower. We have verified this phenomenon via numerical
simulations and also via measurements as discussed later.
Y ( f ) =
T
h
2 j
N
i=1
e
−j (2i−1)πT
h
( f −f
R
i
)
×[Sinc(T
h
( f − f
R
i
− f
T
i
)
×e
j ((2i−1)πT
h
( f
T
i
−f
R
i
)+
T
(i)+
R
(i)
−Sinc(T
h
( f − f
R
i
+ f
T
i
)
×e
−j ((2i−1)πT
h
( f
T
i
−f
R
i
)+
T
(i)+
R
(i)
].
Our measurement results for the transmit signal seen at the
RX channel for a ten-channel separation between the TX and
RX show a 27 dB suppression of the TX signal. For the 8 dBm
TX signal input at the antenna and 20 dB suppression by the
SIC, this results in a −40.4 dBm self-interference in the RX
channel, as shown in Fig. 2. The broadband TX noise signal
can be suppressed in the digital baseband using an axillary path
as in [10] and is not included in this prototype. In the case of
the PA, the primary limitation is that since the TX correlators
are before the PA, the PA needs to be able to pass the fast
hopping signal, i.e., it has to be sufficiently broadband. Not
surprisingly, broadband PAs are normally less power efficient
than high-Q narrow-band PAs.
B. Receive Correlator
The receive correlator consists of two passive four-phase
mixers. N-path mixers use passive switches and a 25% duty
cycle clock generator (for four-phase). These are “digital-
like,” in which they do not have any memory and can
switch frequencies instantly. The only element that maintains
memory is the baseband filter capacitor. As long as the RF
and LO frequencies are synchronized, the memory element
effectively only sees signals close to dc. The mixer on the
left that is synchronized to the received signal de-spreads and
down-converts the desired signal to baseband. At the same
time, the mixer also spreads out any narrow-band blockers
that may exist in-band. The de-spread signal is then filtered
out to remove any out of band interference. In our design,
the de-spreading/processing gain is 20 dB. The blocker is also
reduced by the same amount. The reason for the processing
gain is that the receiver only sees the blocker once every
100 times, effectively reducing its power by a 100 times
or 20 dB. The same thing happens when there are multiple
blockers in-band, i.e., the receiver spreads the blockers such
that the sum of the powers of the resulting spectrums that
show up in the receive channel is 20 dB down. Note that the
spreading and filtering/averaging of the blocker occur in the
current domain, which means that large voltage swings due to
the interferer are avoided.
The resulting baseband signal is then up-converted to a
fixed-frequency RF. As discussed previously, the reason for
this up-conversion is that the front end can be added to
any commercial off-the-shelf (COTS) transceiver, which also
allows us to exploit the excellent noise figure of COTS low
noise amplifier (LNA).
C. Transmit Correlator
The transmit correlator down-converts the fixed-frequency
transmit signal with the mixer to the right and up-converts
it back to RF using the fast-hopping LO signal. Similar
to the receive correlator, the double mixer structure makes
this correlator suitable for use with COTS transceivers. The
fixed-frequency mixers in the receive and transmit correlators
may be removed for an integrated solution.
The hopped transmit signal is then amplified using a broad-
band linear PA. An example broadband PA that meets our
specifications is given in [11]. The PA is operated with 5 dB
backoff to insure linear operation. The 33 dBm PA output
power is fed to the antenna using an off-chip circulator.
This output power enables the transceiver to support a range
of 1.5 Km for the 3 GPP suburban channel model, assuming
that 30 dBm power is radiated from the antenna. The transmit
correlator is placed before the PA to relax its power handling
requirements. However, this means that a high-Q narrow-band
PA may not be used, as it will ring during channel hopping.