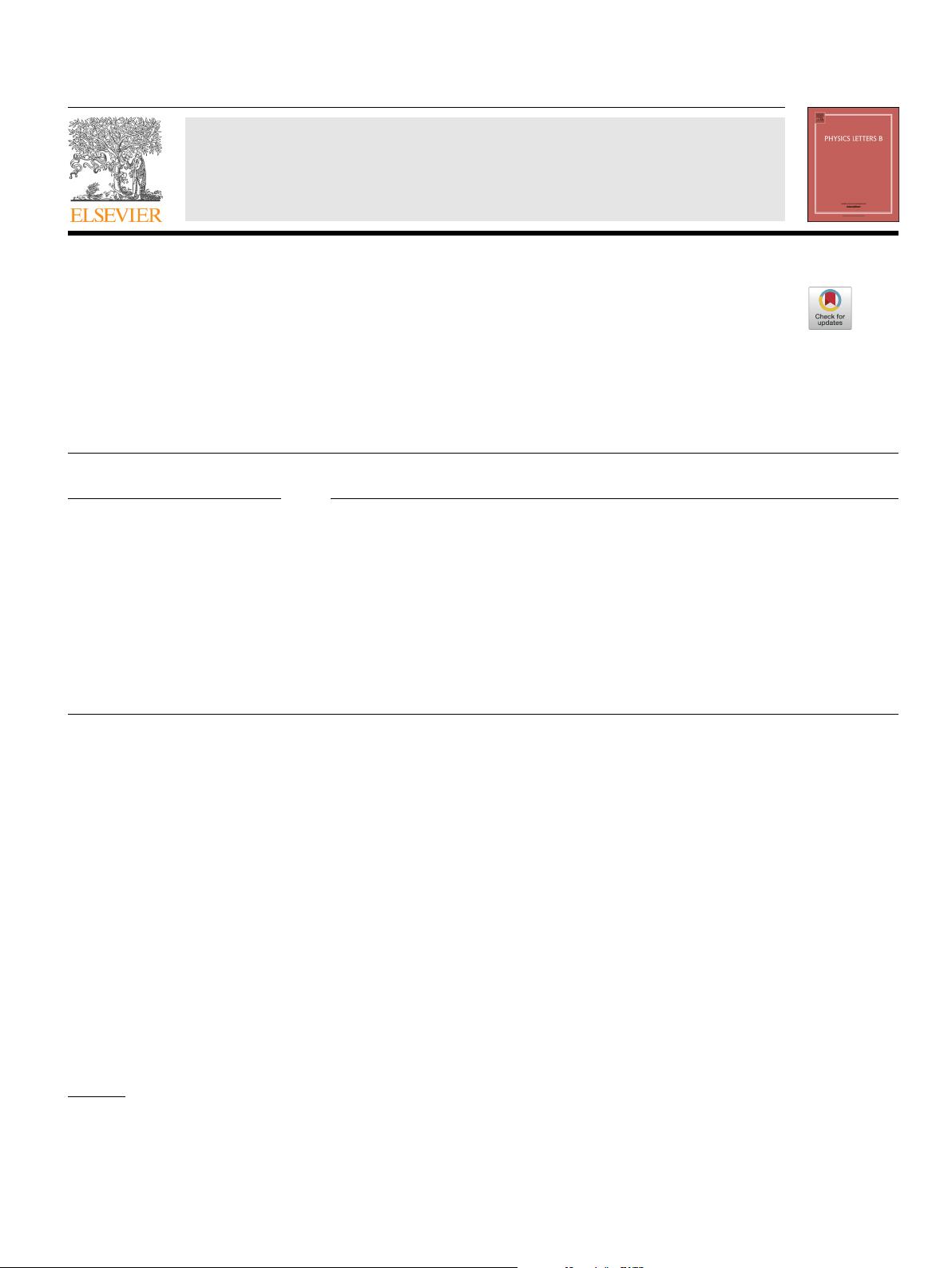
Physics Letters B 780 (2018) 495–500
Contents lists available at ScienceDirect
Physics Letters B
www.elsevier.com/locate/physletb
Hydrodynamic collectivity in proton–proton collisions at 13 TeV
Wenbin Zhao
a,b
, You Zhou
c,∗
, Hao-jie Xu
d,a
, Weitian Deng
e
, Huichao Song
a,b,f,∗
a
Department of Physics and State Key Laboratory of Nuclear Physics and Technology, Peking University, Beijing 100871, China
b
Collaborative Innovation Center of Quantum Matter, Beijing 100871, China
c
Niels Bohr Institute, University of Copenhagen, Blegdamsvej 17, 2100 Copenhagen, Denmark
d
School of Science, Huzhou University, Huzhou 313000, China
e
School of Physics, Huazhong University of Science and Technology, Wuhan 430074, China
f
Center for High Energy Physics, Peking University, Beijing 100871, China
a r t i c l e i n f o a b s t r a c t
Article history:
Received
4 January 2018
Received
in revised form 9 March 2018
Accepted
9 March 2018
Available
online 16 March 2018
Editor:
W. Haxton
In this paper, we investigate the hydrodynamic collectivity in proton–proton (p–p) collisions at 13 TeV,
using iEBE-VISHNU hybrid model with HIJING initial conditions. With properly tuned parameters, our
model simulations can remarkably describe all the measured 2-particle correlations, including integrated
and differential elliptic flow coefficients for all charged and identified hadrons (K
0
S
, ). However, our
model calculations show positive 4-particle cumulant c
2
{4} in high multiplicity pp collisions, and can
not reproduce the negative c
2
{4} measured in experiment. Further investigations on the HIJING initial
conditions show that the fluctuations of the second order anisotropy coefficient ε
2
increases with the
increase of its mean value, which leads to a similar trend of the flow fluctuations. For a simultaneous
description of the 2- and 4- particle cumulants within the hydrodynamic framework, it is required to
have significant improvements on initial condition for pp collisions, which is still lacking of knowledge
at the moment.
© 2018 The Author(s). Published by Elsevier B.V. This is an open access article under the CC BY license
(http://creativecommons.org/licenses/by/4.0/). Funded by SCOAP
3
.
1. Introduction
One of the main goal of the heavy-ion program at Relativistic
Heavy Ion Collider (RHIC) and the Large Hadron Collider (LHC) is
to create a novel state of matter, the Quark-Gluon Plasma (QGP),
and study its properties. The anisotropic flow, that evaluates the
anisotropy of the momentum distribution of final produced par-
ticles,
is sensitive to both initial state fluctuations and the QGP
transport properties [1–7]. Fruitful flow data [8–23] and the suc-
cessfully
descriptions by hydrodynamic calculations [24–31], re-
vealed that
the created QGP fireball behaves like a nearly perfect
liquid with a very small specific shear viscosity η/s close to the
conjectured lowest bound 1/4π [32].
The
high energy proton–lead (p–Pb) and proton–proton (p–p)
collisions at the LHC were originally aimed to provide the refer-
ence
data for the high energy nucleus-nucleus collisions. However,
various unexpected phenomena have been observed in these small
systems, especially in the high multiplicity region. One surpris-
*
Corresponding authors.
E-mail
addresses: you .zhou @cern .ch (Y. Zhou), huichaosong @pku .edu .cn
(H. Song).
ing discovery is the long-range “ridge” structures in two-particle
azimuthal correlations with a large pseudo-rapidity separation in
high multiplicity p–Pb and p–p collisions [33–37]. Such long-range
correlation structures were firstly discovered in Au–Au and Pb–
Pb
collisions and interpreted as a signature of the collective ex-
pansion.
In general, the theoretical interpolations for the long-
range
“ridge” structure in the small systems can be classified into
three big categories: final state interactions, such as hydrodynamic
expansion [38–43], parton cascade [44–47], hadronic rescatter-
ing [48],
rope and shoving mechanism [49], initial state effects
related to the gluon saturation [50–57] and combinations of both
initial and final state effects [58]. For recent theoretical progresses,
please refer to [7,59].
In
experiments, one of the crucial questions on the “ridge”
structure is whether it arises from correlations of all particles re-
lated
to the collective flow or it only involves with the correlations
from few particles, e.g. from resonance decays or jets, which is
defined as non-flow. In small pp and p–Pb systems, the non-flow
contributions are always significant, even in case that collective ex-
pansion
has been developed. It is thus necessary to remove such
non-flow effects before comparing the data with the model cal-
culations.
Based on different assumptions, various non-flow sub-
traction
methods, e.g. template fit [36,60,61] and peripheral sub-
https://doi.org/10.1016/j.physletb.2018.03.022
0370-2693/
© 2018 The Author(s). Published by Elsevier B.V. This is an open access article under the CC BY license (http://creativecommons.org/licenses/by/4.0/). Funded by
SCOAP
3
.