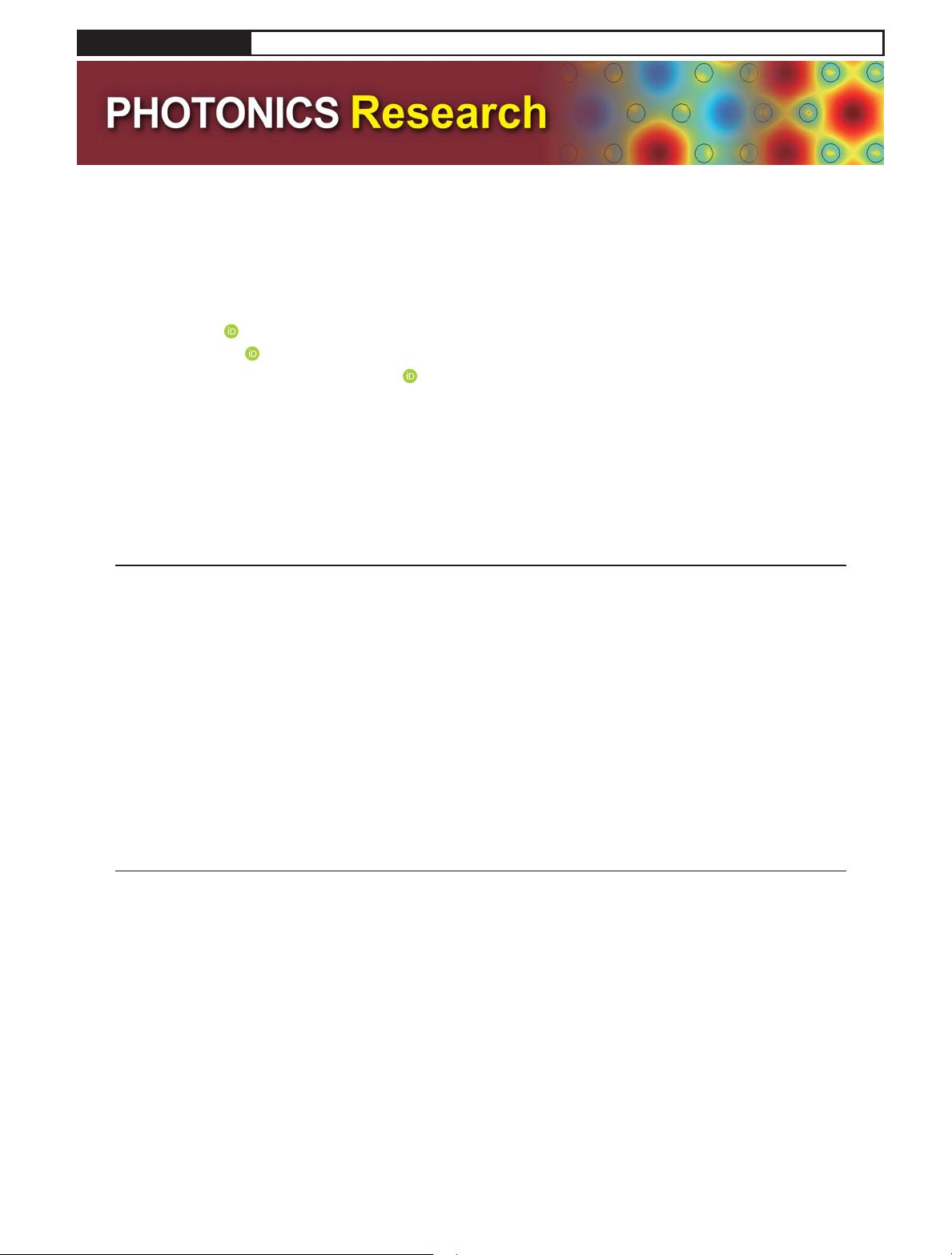
Passively Q-switched femtosecond-laser-written
thulium waveguide laser based on evanescent
field interaction with carbon nanotubes
ESROM KIFLE,
1
PAVEL LOIKO,
2
JAVIER RODRÍGUEZ VÁZQUEZ DE ALDANA,
3
CAROLINA ROMERO,
3
AIRÁN RÓDENAS,
1,4
SUN YUNG CHOI,
5
JI EUN BAE,
5
FABIAN ROTERMUND,
5
VIKTOR ZAKHAROV,
2
ANDREY VENIAMINOV,
2
MAGDALENA AGUILÓ,
1
FRANCESC DÍAZ,
1
UWE GRIEBNER,
6
VALENTIN PETROV,
6
AND XAVIER MATEOS
1,
*
1
Universitat Rovira i Virgili, Departament Quı´mica Fı´sica i Inorgànica, Fı´sica i Cristal·lografia de Materials i Nanomaterials (FiCMA-FiCNA)-EMaS,
Campus Sescelades, E-43007 Tarragona, Spain
2
ITMO University, 49 Kronverkskiy pr., 197101 St. Petersburg, Russia
3
Aplicaciones del Láser y Fotónica, University of Salamanca, 37008 Salamanca, Spain
4
Istituto di Fotonica e Nanotecnologie, Consiglio Nazionale delle Ricerche (IFN-CNR), Piazza Leonardo da Vinci, 32, 20133 Milano, Italy
5
Department of Physics, KAIST, 291 Daehak-ro, Yuseong-gu, 34141 Daejeon, South Korea
6
Max Born Institute for Nonlinear Optics and Short Pulse Spectroscopy, Max-Born-Str. 2a, D-12489 Berlin, Germany
*Corresponding author: xavier.mateos@urv.cat
Received 29 June 2018; revised 17 July 2018; accepted 17 July 2018; posted 17 July 2018 (Doc. ID 336536); published 26 September 2018
Surface channel waveguides (WGs) were fabricated in a monoclinic Tm
3
:KLuWO
4
2
crystal by femtosecond
direct laser writing (fs-DLW). The WGs consisted of a half-ring cladding with diameters of 50 and 60 μm located
just beneath the crystal surface. They were characterized by confocal laser microscopy and μ-Raman spectroscopy,
indicating a reduced crystallinity and stress-induced birefringence of the WG cladding. In continuous-wave (CW)
mode, under Ti:sapphire laser pumping at 802 nm, the maximum output power reached 171.1 mW at 1847.4 nm,
corresponding to a slope efficiency η of 37.8% for the 60 μm diameter WG. The WG propagation loss was
0.7 0.3dB∕cm. The top surface of the WGs was spin-coated by a polymethyl methacrylate film containing
randomly oriented (spaghetti-like) arc-discharge single-walled carbon nanotubes serving as a saturable absorber
based on evanescent field coupling. Stable passively Q-switched (PQS) operation was achieved. The PQS
60 μm diameter WG laser generated a record output power of 150 mW at 1846.8 nm with η 34.6%. The
conversion efficiency with respect to the CW mode was 87.6%. The best pulse characteristics (energy/duration)
were 105.6 nJ/98 ns at a repetition rate of 1.42 MHz.
© 2018 Chinese Laser Press
OCIS codes: (230.7380) Waveguides, channeled; (140.3540) Lasers, Q-switched; (140.3380) Laser materials.
https://doi.org/10.1364/PRJ.6.000971
1. INTRODUCTION
Waveguide (WG) lasers emitting in the spectral range of ∼2 μm
are of interest for bio- and environmental sensing applications.
This is because such a radiation matches spectrally with the ab-
sorption of some relevant molecules, such as H
2
O or CO
2
.
Typically, laser emission at ∼2 μm is achieved using thulium
(Tm
3
) or holmium (Ho
3
) ions. In the former case, the emis-
sion is due to the
3
F
4
→
3
H
6
transition [1]. Tm
3
ions are at-
tractive because of a strong absorption at ∼0.8 μm (
3
H
6
→
3
H
4
transition), efficient cross-relaxation (CR) enhancing the pump
quantum efficiency up to 2 [2], low-threshold behavior, and
typically large Stark splitting of the ground state leading to a
broadband tuning feature.
Highly efficient laser operation was achieved with
continuous-wave (CW) Tm WG lasers. In Ref. [3], a “mixed”
Tm:KY, Lu, GdWO
4
2
crystalline channel WG laser gener-
ated 1.6 W at 1.84 μm with a record slope efficiency η of 80%,
promoted by an efficient CR at a high Tm
3
doping level.
The active layer was fabricated by liquid phase epitaxy (LPE)
[4]. The WG propagation loss δ was ∼0.1dB∕cm [5]. The
refractive index contrast with respect to the undoped
KYWO
4
2
substrate Δn was ∼1 × 10
−3
. Note that the active
material belongs to the crystal family of monoclinic double
tungstates (DTs), which are well-known for Tm
3
ion doping
due to their advantageous spectroscopic properties for polarized
light [6].
Research Article
Vol. 6, No. 10 / October 2018 / Photonics Research 971
2327-9125/18/100971-10 Journal © 2018 Chinese Laser Press