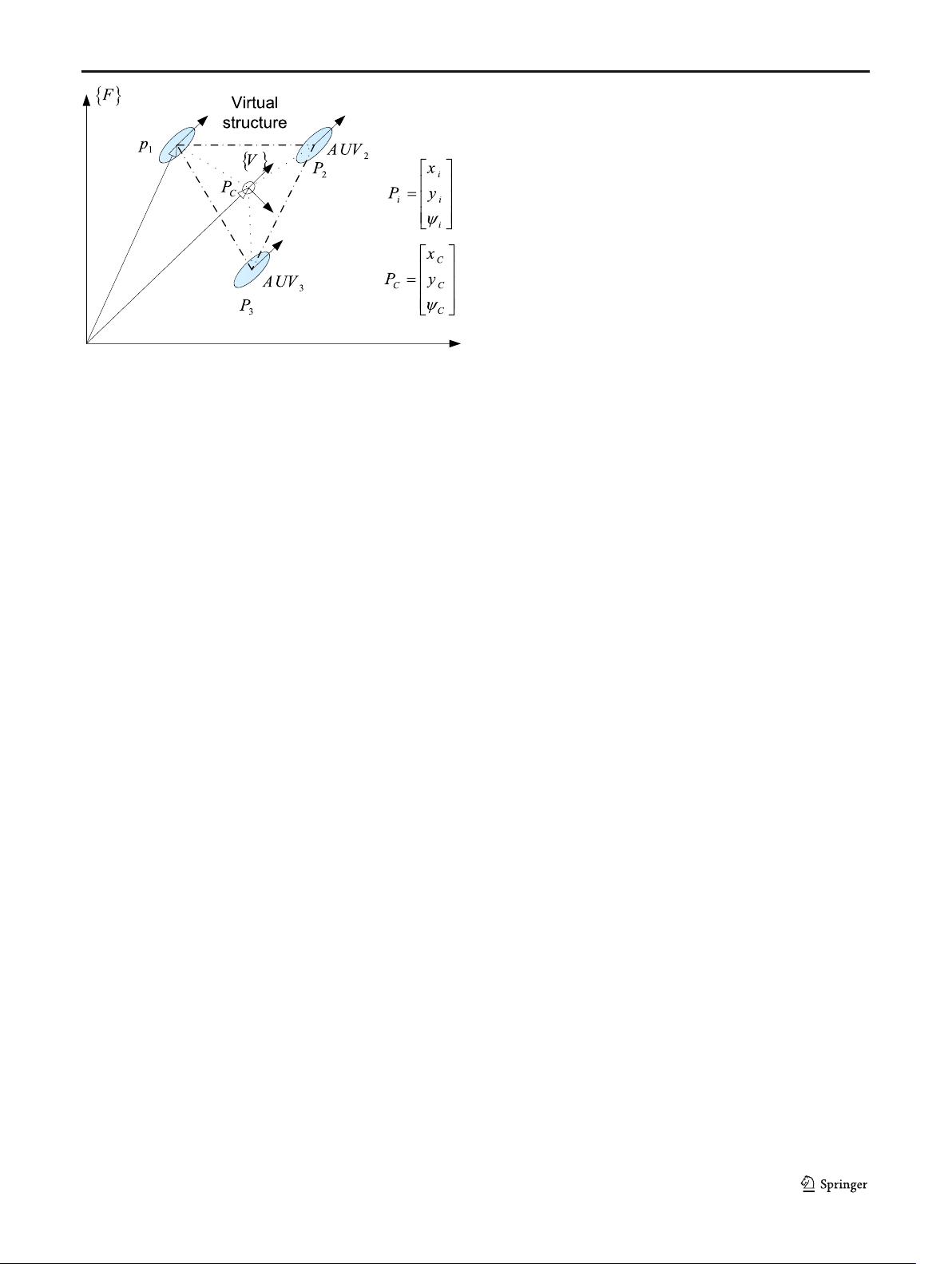
considered equal to the number of AUVs and one-
to-one correspond ence is established between
them. The desired formation is obtained by mini-
mizing the position error function, which is defined
based on the current position of the AUVs and their
desired position in the virtual structure.
Stepe 2: Converting the virtual structure’s desired motion
into desired commands for each of the AUVs in
the group: Having adjusted the desired virtual
structure, a virtual force is applied to the virtual
structure so that the structure can be moved to the
next point. In this section, the vehicle’smotionca-
pacity is taken into consideration.
Stepe 3: Designing an individual tracking controller for each
of the AUVs: After transferring the virtual structure
to the new position (done in Step 2), the control
inputs for each vehicle are designed in such a way
that the vehicle moves to its new corresponding
point in the virtual structure. A simple way for the
movement of vehicles to the desired position is
changing direction towards the desired position
and then transferring to the target point.
Virtual structure is usually used for formation control in
mobile robots, spacecraft [42–44] or small satellites [11,
45–47]. The employment of virtual structure approac h in
spacecraft formation control was presented in [48], where for-
mation feedback from the spacecraft to the virtual structure
was consulted. This would give robustness to the structure of
formation against internal or external disturbances and lead to
better performance. The control strategies of the distributed
virtual structure along with the corresponding coupling of
single-wheeled mobile robots are introduced in [49]. The cor-
responding coupling would create a trade-off between ensur-
ing the tracking of each robot’s desired trajectories and main-
taining the shape of the formation when faced with
disturbances, which can result in higher robustness of the pro-
posed formation structure. The exponential stability of the
dynamics of formation error is proven and the proposed con-
trol design is practically applied. In [50], the two-layer distrib-
uted formation learning control was presented for one group
of AUVs, which included an adaptive distributed observer in
the upper layer and a decentralized deterministic learning con-
troller in the lower layer. The upper layer estimates the virtual
leader’s states and dynamics. The formation tracking control
and learning performance of the neural network with local
information are done in the lower layer.
3.3 Formation Control Based on Behavioral Structure
Behavior-based formation control was first introduced by
Balch and Arkin in formation control [51]. This method uses
a weighted hybrid of different mission objectives in order to
generate vehicle control inputs. Generally, control targets in-
clude maintaining desired formation, moving towards the tar-
get, avoiding obstacle collisions, and avoiding inter-group
collisions. Each of the aforementioned items is prioritized
with different gains and the obtained average is given to each
vehicle as a control input. Meeting several control objectives
simultaneously is one of the benefits of this approach. It is,
however, hard to describe the dynamic of the group and guar-
antee the stability of the whole system, because the kinematic
and dynamic features of the vehicle are not taken into consid-
eration. The behavioral structure method is commonly applied
in combination with the artificial potential field method. In
[52], the formation control of a swarm of robots is considered
on the basis of the behavioral method for the generation of
formation and obstacles avoidance. To create an initial forma-
tion, the classification-based target searching algorithm is
used so that any arbitrary shape can be generated. Moreover,
the behavior-based approach is employed for moving in an
unknown obstacle-strewn environment. Based on the non-
linear dynamic systems theory, the distributed formation con-
trol and obstacle avoidance are presented in [53]. The desired
trajectories are gained based on the behavioral variables’ state
space. A combination of behavior-based formation approach
and artificial potential field is one of the most common
methods of formation control, which is also used for control-
ling a group of AUVs with simplified second-order dynamic
equation. The potential function for each vehicle is defined in
terms of goals, obstacles and formation structures and the
control law is designed based on Lyapunov’sdirectmethod.
This will ensure that the AUVs will effectively follow the goal
and avoid obstacles [54]. The combination of virtual and be-
havioral structures is the focal point of other studies conducted
on combining different methods of formation control. This
approach is used for the formation control of a group of mo-
bile robots. Each robot is modeled by an electric charge. Upon
defining the attraction forces, the robots would move towards
Fig. 4 The virtual structure including three vehicles
J Intell Robot Syst (2021) 101:
Page 5 of 26 67