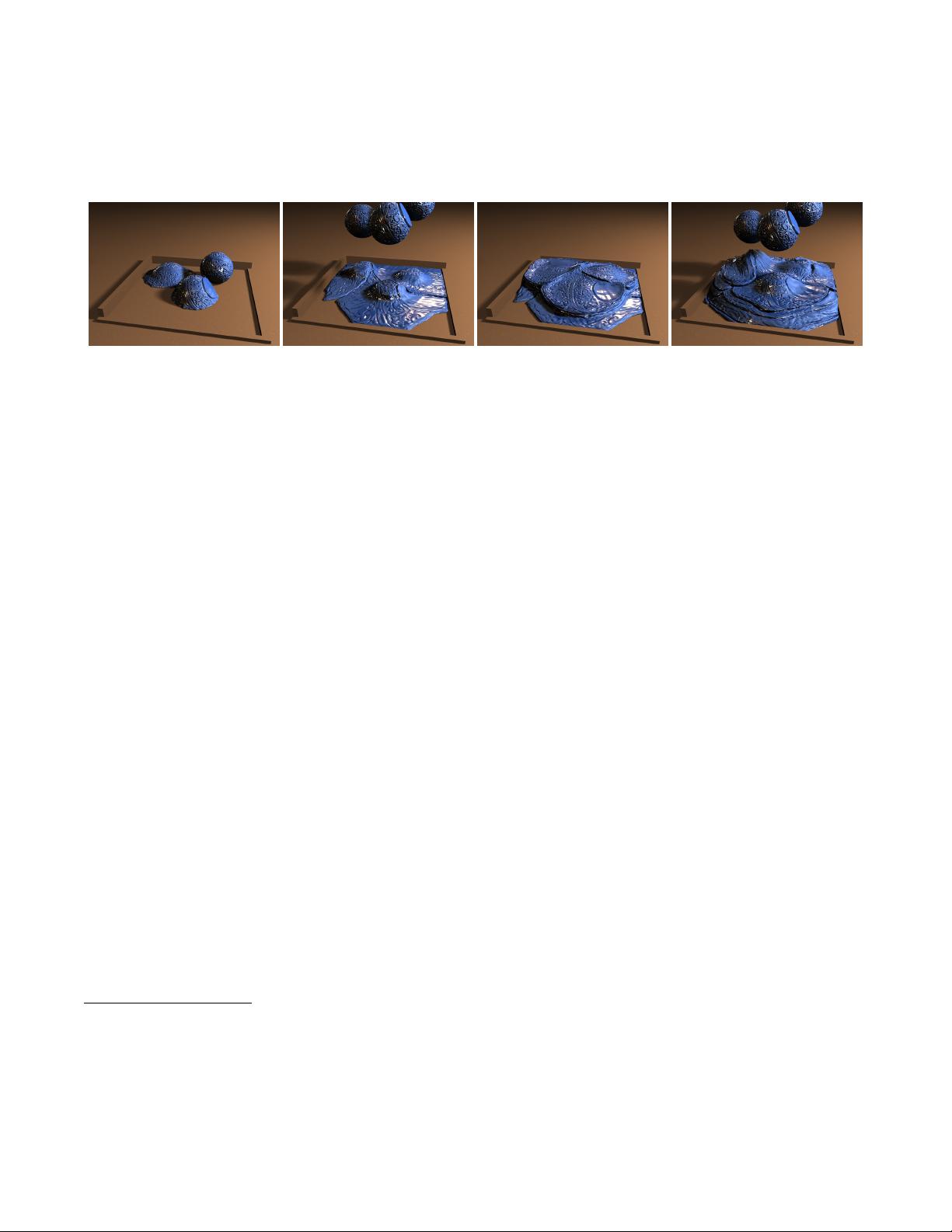
Deforming Meshes that Split and Merge
Chris Wojtan Nils Th
¨
urey Markus Gross Greg Turk
Georgia Institute of Technology ETH Z
¨
urich ETH Z
¨
urich Georgia Institute of Technology
Figure 1: Dropping viscoelastic balls in an Eulerian fluid simulation. Invisible geometry is quickly deleted, while the visible surfaces retain
their details even after translating through the air and splashing on the ground.
Abstract
We present a method for accurately tracking the moving surface of
deformable materials in a manner that gracefully handles topologi-
cal changes. We employ a Lagrangian surface tracking method, and
we use a triangle mesh for our surface representation so that fine
features can be retained. We make topological changes to the mesh
by first identifying merging or splitting events at a particular grid
resolution, and then locally creating new pieces of the mesh in the
affected cells using a standard isosurface creation method. We stitch
the new, topologically simplified portion of the mesh to the rest of
the mesh at the cell boundaries. Our method detects and treats topo-
logical events with an emphasis on the preservation of detailed fea-
tures, while simultaneously simplifying those portions of the ma-
terial that are not visible. Our surface tracker is not tied to a par-
ticular method for simulating deformable materials. In particular,
we show results from two significantly different simulators: a La-
grangian FEM simulator with tetrahedral elements, and an Eulerian
grid-based fluid simulator. Although our surface tracking method is
generic, it is particularly well-suited for simulations that exhibit fine
surface details and numerous topological events. Highlights of our
results include merging of viscoplastic materials with complex ge-
ometry, a taffy-pulling animation with many fold and merge events,
and stretching and slicing of stiff plastic material.
Keywords: Physically Based Animation, Deformable Meshes,
Fluid Simulation, Topological Control
1 Introduction
The physical world is rich in materials that deform. In many in-
stances, these materials easily undergo topological changes, allow-
ing separation or joining of components. Such materials include
water, toothpaste, bread dough, peanut butter, taffy, tar, and clay. In
order to produce plausible animations of these deforming materials,
we must develop simulation techniques that retain visual details, but
at the same time allow topological changes to the surface. This is
the focus of our work.
We introduce a method of tracking and updating the surface of
a deforming object in a manner that gracefully allows for topology
changes. Our approach uses a mesh to represent the surface of ob-
jects. The key benefit of using a mesh is that it allows the surface to
be moved in a Lagrangian manner, using a high-order ODE integra-
tor to move the vertices of the surface. This results in the retention
of fine surface details. The drawback of using a mesh surface is that
changing the topology of a mesh is notoriously tricky, both in terms
of robustness and program complexity. Our approach avoids these
difficulties by replacing those portions of the mesh where a topol-
ogy change should occur with a simplified surface that is generated
using a standard isosurface creation method. This new portion of
the mesh is then stitched together with the untouched parts of the
surface.
Our approach to topology change lets us decide which potential
topological modifications are permitted and which ones should be
prevented. This allows us to customize the behavior of the simu-
lator in order to simulate materials with particular attributes. For
example, we can allow surface separation events, but forbid surface
merging. This might be appropriate for simulating bread dough that
has been rolled in flour so that it no longer sticks to itself. One par-
ticular rule for marking where a merge event should be triggered
is what we refer to as the deep cell test. This test allows surface
merging when it is deeply embedded in the folds of a material, but
it leaves alone potential merge events that are near the surface and
that might produce visually distracting results.
The key attributes of our approach to surface tracking are as fol-
lows:
• Our algorithm performs topological splits and merges, which
decreases the memory and computation requirements of deal-
ing with complicated surfaces and permits interesting behav-
iors like the merging of water droplets.
• Our simulator retains thin features and fine surface details.
• The method is robust, even during complex topology changes.
• The surface tracker can be easily tuned to allow or forbid par-
ticular kinds of topology changes to better suit a given mate-
rial.
• Our surface tracker does not depend upon any particular sim-
ulation technique. In particular, we demonstrate results from
both an Eulerian fluid solver and an FEM simulator.