【Introduction】: Demystifying the Principles of Generative Adversarial Networks (GANs): Essential Basics and Techniques for Beginners
发布时间: 2024-09-15 16:22:41 阅读量: 26 订阅数: 26 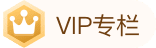
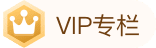
# 1. Introduction to Generative Adversarial Networks (GANs): Essential Basics and Techniques for Beginners
Generative Adversarial Networks (GANs) represent a significant breakthrough in the field of deep learning. They consist of two neural networks—the generator and the discriminator—which compete and promote each other's improvement throughout the training process. The generator aims to produce counterfeit data that is as close to real data as possible, while the discriminator's goal is to accurately differentiate between real data and the fake data produced by the generator. This adversarial process enables GANs to generate high-quality synthetic data such as images, audio, and video. The emergence of GANs has led to new breakthroughs in AI fields like image generation, image editing, and data augmentation, offering new possibilities for the advancement of AI.
# 2. Theoretical Foundations and Mathematical Principles of GANs
### 2.1 Structure and Workflow of GANs
#### 2.1.1 Role and Principle of the Generator
The generator is a core component of the GAN, primarily tasked with generating realistic data samples based on input random noise vectors. Specifically, the generator maps the random noise to the data space through a deep neural network, aiming to make the generated data samples as similar as possible to the real ones, to the point of being indistinguishable. To achieve this goal, the generator needs to learn the distribution of real data and reproduce the statistical characteristics of the data based on the noise input.
Mathematically, let's assume the distribution of the real data sample is \(P_{data}\), the goal of the generator is to learn a mapping function \(G\) that can map a noise vector \(z\) to a sample \(x\) in the data space \(X\). Therefore, the generator \(G\) can be seen as a mapping from the noise distribution \(P_z\) to the data distribution \(P_{data}\). In practice, deep neural networks such as fully connected networks or convolutional neural networks are often used to implement \(G\)'s mapping function.
In programming, the construction of the generator typically employs deep learning frameworks such as TensorFlow or PyTorch. The following is a simplified pseudo-code of a generator model, implemented using the PyTorch framework:
```python
import torch
import torch.nn as nn
class Generator(nn.Module):
def __init__(self, input_dim, hidden_dim, output_dim):
super(Generator, self).__init__()
self.fc = nn.Sequential(
nn.Linear(input_dim, hidden_dim),
nn.ReLU(),
nn.Linear(hidden_dim, hidden_dim),
nn.ReLU(),
nn.Linear(hidden_dim, output_dim),
nn.Tanh()
)
def forward(self, x):
return self.fc(x)
```
In this example, the `input_dim` represents the dimension of the noise vector, `hidden_dim` represents the dimension of the hidden layers, and `output_dim` is the target dimension for the generated data samples. The generator uses three fully connected layers and ReLU activation functions, with the output layer using the Tanh activation function to ensure that the output data is within the (-1, 1) range, facilitating subsequent processing.
The design of the generator needs to balance the model's capacity and the stability of training. A network structure that is too simple may fail to capture the true data distribution, while a structure that is too complex may lead to difficulties in training or even mode collapse issues. Therefore, when designing the generator, it is necessary to weigh the complexity of the network against its generalization ability.
#### 2.1.2 Function and Mechanism of the Discriminator
The discriminator is equally indispensable in the GAN. Its main function is to classify data samples, distinguishing which samples come from the real data distribution and which are fake data generated by the generator. By learning the differences between real and generated samples, the discriminator assigns a probability score indicating the likelihood that the input sample is real. Therefore, the discriminator's objective function is to maximize its ability to correctly classify the two types of samples.
Mathematically, the discriminator \(D\) is considered a binary classifier whose goal is to learn a function \(D(x)\) that can output the probability that a given data sample \(x\) comes from the real data distribution \(P_{data}\). The closer this probability is to 1, the more likely the discriminator considers the sample to be real; conversely, the closer it is to 0, the more likely the sample is considered fake, generated by the generator.
In programming, the structure of the discriminator typically also uses a deep neural network, which can be a convolutional neural network (CNN) or a fully connected network. The following is a simplified pseudo-code of a discriminator model, implemented using the PyTorch framework:
```python
class Discriminator(nn.Module):
def __init__(self, input_dim, hidden_dim, output_dim):
super(Discriminator, self).__init__()
self.fc = nn.Sequential(
nn.Linear(input_dim, hidden_dim),
nn.LeakyReLU(0.2),
nn.Linear(hidden_dim, hidden_dim),
nn.LeakyReLU(0.2),
nn.Linear(hidden_dim, output_dim),
nn.Sigmoid()
)
def forward(self, x):
return self.fc(x)
```
In this example, we also use three fully connected layers and LeakyReLU activation functions. The output layer uses the Sigmoid activation function to compress the output value into the (0, 1) range, representing the probability of the input data being a real sample.
To train the discriminator, we need to sample from the real data set and also use the fake data generated by the generator. The discriminator's loss function is usually calculated based on the cross-entropy loss, with the goal of maximizing the accuracy of identifying real samples while minimizing the accuracy of identifying fake samples. Through this process, the discriminator continues to optimize, enhancing its鉴别 (discernment) ability.
### 2.2 Loss Functions and Optimization Processes
#### 2.2.1 Design and Significance of Loss Functions
In GANs, both the generator and the discriminator are improved by optimizing a loss function. The loss function is crucial for the training of GANs because it not only provides the optimization objective for both the generator and discriminator but also affects the stability of the entire GAN training and the quality of the final generated samples.
In the basic setup of GANs, the loss functions for the generator \(G\) and discriminator \(D\) are as follows:
- The loss function for the generator \(G\), \(L_G\):
\[L_G = -\log D(G(z))\]
Here, \(D(G(z))\) is the real probability that the discriminator assigns to the generated data \(G(z)\). Since \(D\) is a probability output, \(-\log D(G(z))\) is effectively maximizing the probability that \(G\) generates data that is misjudged as real. In practice, sometimes \(\log(1 - D(G(z)))\) is used, especially when \(D(G(z))\) approaches 1, to avoid gradient vanishing issues.
- The loss function for the discriminator \(D\), \(L_D\):
\[L_D = -\log D(x) - \log(1 - D(G(z)))\]
Here, the first term \(-\log D(x)\) is maximizing the correct classification probability for real data by \(D\), and the second term \(-\log(1 - D(G(z)))\) is maximizing the correct classification probability for fake data. Therefore, \(L_D\) is maximizing the discrimination ability for both real and fake data simultaneously.
This loss function design is based on the idea of game theory, where the generator and discriminator will engage in a mutual game during training, eventually reaching a dynamic equilibrium. However, in practice, this original GAN loss function often leads to unstable training and can easily result in mode collapse issues. Therefore, researchers have proposed many improved loss functions, such as the Wasserstein loss and the LSGAN loss, to improve the GAN training process.
#### 2.2.2 Application of Optimization Algorithms in GANs
Training GANs is a non-convex optimization problem, and due to its unique adversarial structure, the training process is often more complex and challenging than ordinary deep learning models. Therefore, selecting an appropriate optimization algorithm and adjusting its parameters are crucial for successfully training GANs.
A key challenge in training GANs is how to balance the training speed of the generator and discriminator. If the discriminator is too strong, it may prematurely reject the samples generated by the generator, preventing the generator from receiving sufficient gradient updates and effectively learning. Conversely, if the generator is too strong, the discriminator may find itself unable to distinguish between real and fake data, leading to training failure. Therefore, it is necessary to adjust the update frequency of the generator and discriminator during training, also known as learning rate scheduling strategies, to ensure the balance between the two.
In terms of choosing optimization algorithms, gradient descent methods and their variants (such as Adam, RMSprop, etc.) are the most common choices. These optimization algorithms achieve the minimization of the loss function by adjusting the update step size for each parameter. In the training of GANs, it is usually necessary to choose different optimizers for the generator and discriminator or to set different learning rates for both.
The following is a simple training process pseudo-code example, which uses the Adam optimizer:
```python
# Set the optimizers for the generator and discriminator
optimizer_G = torch.optim.Adam(G.parameters(), lr=0.0002, betas=(0.5, 0.999))
optimizer_D = torch.optim.Adam(D.parameters(), lr=0.0002, betas=(0.5, 0.999))
# Training process
for epoch in range(num_epochs):
for i, data in enumerate(dataloader, 0):
# Actual data
real_data = data
# Generate noise
z = torch.randn(batch_size, noise_dim)
# Train the discriminator
optimizer_D.zero_grad()
# Calculate the loss for real data
real_data_loss = loss_fn(D(real_data), 1)
# Calculate the loss for fake data
fake_data = G(z)
fake_data_loss = loss_fn(D(fake_data.detach()), 0)
# Backpropagation
d_loss = real_data_loss + fake_data_loss
d_loss.backward()
optimizer_D.step()
# Train the generator
optimizer_G.zero_grad()
# Calculate the generator's loss
output = D(fake_data)
g_loss = loss_fn(output, 1)
# Backpropagation
g_loss.backward()
optimizer_G.step()
```
In this training process, the parameters of the generator are frozen first in each iteration, and only the discriminator's parameters are updated, followed by updating the generator's parameters. This strategy is known as "discriminator first, generator second" and helps to balance the learning process for both. Note that, to avoid gradient vanishing or explosion, different strategies are usually employed to update the generator and discriminator. For example, during backpropagation, the generator can freeze the discriminator's parameters to ensure that the training of the generator is not disturbed.
### 2.3 Theoretical Advancement: Mode Collapse and Its Solutions
#### 2.3.1 Definition and Impact of Mode Collapse
Mode collapse is a common failure phenomenon in the training of generative adversarial networks, where the generator falls into a local optimum and begins to repeatedly generate one or a few similar or identical samples, leading to a sharp decrease in the diversity of the generated samples. When the discriminator can easily identify these fake samples, the generator will not receive effective gradient signals and will not be able to continue learning, ultimately resulting in training failure for the entire model.
The causes of mode collapse are multifaceted, possibly because the generator cannot capture the true distribution of data, or the discriminator is too powerful, preventing the generator from learning enough information to improve the generated data. In addition, inappropriate design of the GAN loss function or improper choice of optimization algorithms can also lead to mode collapse.
The impact of mode collapse is that it destroys the diversity of the generator, causing the results of the model to lack variability. In severe cases, the entire GAN training process cannot continue. Therefore, preventing mode collapse is an important topic in GAN research.
#### 2.3.2 Methods to Prevent and Solve Mode Collapse
To address the problem of mode collapse, researchers have proposed various strategies and methods, with the following being some of the main solutions:
1. **Use Wasserstein Loss Function**: Wasserstein loss can alleviate the problems caused by the loss function in traditional GANs and provide more stable gradients, helping to reduce mode collapse. GANs based on Wasserstein loss are known as WGANs.
2. **Gradient Penalty Techniques**: For example, WGAN-GP (WGAN with Gradient Penalty), by adding an additional gradient penalty term to the loss function, ensures that the generated distribution has a certain smoothness, thereby alleviating the mode collapse phenomenon.
3. **Introduce Diversity**: By introducing noise or using diverse training samples, the generator can learn richer and more diverse data representations, reducing the risk of mode collapse.
4. **Minimax Strategy**: Use various loss functions and optimization strategies, such as Least Squares GAN (LSGAN), to avoid mode collapse by minimizing the error between real data and fake data.
5. **Feature Matching**: Match the features of the samples generated by the generator to those of the real samples at certain intermediate layers, thereby increasing the diversity of the generated samples.
6. **Regularization Techniques**: Introduce regularization terms to constrain the learning process of the generator or discriminator, avoiding overfitting or fitting to specific samples.
By combining these strategies, the phenomenon of mode collapse can be significantly alleviated, improving the training process of GANs and the quality of the generated samples. However, mode collapse remains an active research area, and methods to solve this problem are also constantly evolving.
# 3. Practical Operations of GANs
## 3.1 Common GAN Architectures and Variants
### 3.1.1 Principles and Implementation of DCGAN
The Deep Convolutional Generative Adversarial Network (DCGAN) is a variant of GAN that incorporates the powerful feature extraction capabilities of CNNs, significantly improving the quality of image generation. DCGAN replaces fully connected layers with convolutional layers in traditional GANs, improving the network structure, allowing GANs to achieve significant performance improvements in image generation tasks. The following are some of the key design principles of DCGAN:
- **Use of Convolutional Layers**: Utilize transposed convolutional layers for upsampling, replacing the fully connected layers in GANs to allow the model to process the hierarchical structure of images.
- **Batch Normalization**: Introduce batch normalization in the layers of the generator and discriminator to stabilize the training process and improve the quality of results.
- **Removal of Fully Connected Layers**: Except for the output and input layers, all other layers in DCGAN use convolutional layers to preserve the two-dimensional structure of images.
- **Activation Functions**: Use LeakyReLU and tanh activation functions to enhance the model's non-linear capabilities and avoid gradient vanishing issues.
During the implementation of DCGAN, we can use deep learning frameworks like PyTorch to build the generator and discriminator networks. The following is a simplified example of DCGAN generator and discriminator code blocks implemented using PyTorch:
```python
import torch
import torch.nn as nn
# Define the generator
class DCGAN_Generator(nn.Module):
def __init__(self, input_dim):
super(DCGAN_Generator, self).__init__()
self.main = nn.Sequential(
# Upsampling and convolutional operations
nn.ConvTranspose2d(input_dim, 1024, 4, 1, 0, bias=False),
nn.BatchNorm2d(1024),
nn.ReLU(True),
# ... Other layer definitions
nn.ConvTranspose2d(128, 3, 4, 2, 1),
nn.Tanh()
)
def forward(self, input):
return self.main(input)
# Define the discriminator
class DCGAN_Discriminator(nn.Module):
def __init__(self):
super(DCGAN_Discriminator, self).__init__()
self.main = nn.Sequential(
# Convolutional operations
nn.Conv2d(3, 64, 4, 2, 1, bias=False),
nn.LeakyReLU(0.2, inplace=True),
# ... Other layer definitions
nn.Conv2d(128, 1, 4, 1, 0, bias=False),
nn.Sigmoid()
)
def forward(self, input):
return self.main(input)
```
In implementation, parameters such as the input dimension `input_dim`, number of network layers, and number of convolutional kernels need to be adjusted based on the actual situation of the dataset. By training the generator and discriminator described above, DCGAN can generate high-quality images.
### 3.1.2 Introduction to Other GAN Architectures (e.g., WGAN, StyleGAN)
In addition to DCGAN, there are many other variants that have proposed unique solutions to different problems. For example, the Wasserstein Generative Adversarial Network (WGAN) introduces the Wasserstein distance to measure the difference between real and generated images, effectively solving the problem of mode collapse during training. StyleGAN, on the other hand, introduces the concept of style control, allowing for higher diversity and quality in the generated images.
#### WGAN
WGAN is a variant of GAN that measures the difference between the generated distribution and the real distribution using the Earth-Mover (also known as Wasserstein-1) distance. Its core idea is to use a parameterized neural network (discriminator) to approximate the Wasserstein distance between the two distributions. The improvements of WGAN include:
- **Use of Weight Clipping**: Limit the range of network weights to prevent excessively large weight updates and maintain gradient stability.
- **Use of Wasserstein Distance**: Train the discriminator to be an approximation of the Wasserstein distance between the generated and real images.
- **Removal of Batch Normalization**: Avoid using batch normalization layers in the discriminator to prevent the introduction of constraints.
#### StyleGAN
StyleGAN introduces the innovative concept of injecting style information into the generator, allowing for fine control over the high-level features and textures of the generated images. The key features of StyleGAN include:
- **Mapping Network**: Transforms the input from the latent space into intermediate latent codes.
- **Adaptive Instance Normalization (AdaIN)**: Used to combine style codes, controlling the style of the generated images.
- **Multi-scale Synthesis**: Gradually synthesizes images at different resolutions, ultimately producing high-resolution output.
Each GAN architecture has been optimized for specific challenges and requirements. They have advanced the application of GANs in various fields through innovative network structures and training techniques.
## 3.2 Practical Tips: Considerations for Training GANs
### 3.2.1 Data Preprocessing and Augmentation Methods
Before training a GAN, data preprocessing is a crucial step. High-quality input data can significantly improve the quality of the generated images. The following are some common data preprocessing and augmentation methods:
- **Normalization**: Normalize the pixel values of images to a smaller range, such as [0,1] or [-1,1], which helps with the convergence of the model and the stability of training.
- **Standardization**: Calculate the mean and standard deviation of the entire dataset and standardize the image data, making it more aligned with a normal distribution.
- **Data Augmentation**: Generate new training samples through operations such as rotation, scaling, and cropping to increase the diversity of samples and enhance the generalization ability of the model.
### 3.2.2 Monitoring and Debugging During the Training Process
The training process of GANs is complex and prone to issues such as non-convergence or mode collapse. Therefore, real-time monitoring of the training process and debugging the model are crucial. The following are some common monitoring and debugging methods:
- **Visualization of Loss Functions**: Plot the loss function curves of the generator and discriminator to observe whether the model is converging.
- **Visualization of Generated Samples**: Periodically generate image samples to intuitively assess the quality and diversity of the generated images.
- **Adjusting Learning Rate and Batch Size**: If problems arise during training, they can be improved by adjusting the learning rate or batch size.
- **Anomaly Detection**: Monitor the occurrence of anomalies, such as a sudden rise or fall in the discriminator's loss, which may indicate that there is a problem with training.
In practice, combining specific application scenarios, choosing appropriate preprocessing and augmentation techniques, as well as appropriate monitoring and debugging strategies, can help us better control the GAN training process and achieve satisfactory training results.
## 3.3 Case Studies of Generating High-Quality Images
### 3.3.1 Applications in Super Resolution and Image Inpainting
Super resolution (SR) and image inpainting are two important applications of GANs in the field of image processing. Through training, GANs can enhance the resolution of images while preserving their content or fill in missing parts of images.
#### Super Resolution
The high-resolution images generated by GANs through super resolution can significantly improve the visual quality of images. For example, using the SRGAN (Super Resolution GAN) model, low-resolution images can be converted into clear high-resolution versions. The core structure of SRGAN includes:
- **Generator**: Utilizes a residual network (ResNet) structure to improve the learning ability of the network.
- **Discriminator**: Designed as a classifier to distinguish between generated images and real high-resolution images.
In implementing SRGAN, the generator progressively放大 the image to the required resolution while preserving the image's key features and details. The discriminator guides the generator's improvements by comparing the generated images with real images.
#### Image Inpainting
The image inpainting task involves filling in holes or damaged parts of images using GANs. A typical model is PGGAN (Progressive GAN), which progressively trains, gradually increasing the depth and resolution of both the generator and discriminator. In the image inpainting scenario, the generator needs to learn how to predict the content of missing parts based on the known parts of the image to produce natural visual effects.
### 3.3.2 Applications in Image Style Transfer and Artistic Processing
Image style transfer is the process of converting a content image into a specific artistic style. GANs excel in this application, especially in style migration and artistic image processing. For example, by using neural style transfer technology, Van Gogh's painting style can be applied to any given image. The fundamental idea of this technology is:
- **Content Image**: Maintain the structural features of the content image.
- **Style Image**: Extract the texture and color features of the style image.
- **Optimization Process**: Through the optimization process, adjust the generated image so that its content comes from the content image, while the style comes from the style image.
In practice, neural networks (such as VGG networks) are used to extract features, and the optimization is based on gradient descent methods. With this technology, GANs can, while preserving the image's content, impart new artistic styles to images, creating creative works.
The above cases demonstrate the application of GANs in generating high-quality images, proving their strong capabilities and potential in the field of image processing through these practices.
# 4. Applications of GANs in Different Fields
## 4.1 Examples of GAN Applications in Image Processing
### 4.1.1 Face Recognition and Image Segmentation
Face recognition technology has been greatly enhanced through the powerful capabilities of GANs. GANs can generate large datasets of high-quality face images, which are particularly important when data is limited. Additionally, GANs can transform images to create new, untagged samples to enhance the diversity and depth of training sets. For example, using the CycleGAN architecture, one can convert one face image into another, thereby assisting in training face recognition algorithms to improve their accuracy and robustness.
In the field of image segmentation, GANs also show their unique advantages. Through the generator, GANs can produce precise image segmentation masks, assisting in segmentation tasks with fewer labeled data. For example, in medical image segmentation, GANs can generate realistic images of lesion areas, aiding medical experts in annotation and thereby improving the accuracy of image segmentation models.
### 4.1.2 Image-to-Image Translation
GANs have widespread applications in image-to-image (image-to-image) translation tasks, involving the transformation of one type of image into another, such as converting satellite images into map views or sketches into real scene photos. Models such as CycleGAN and Pix2Pix are representatives in this field. These models learn the mapping relationship between different image domains through training and can complete complex style transfer tasks.
In image-to-image translation, the design of the generator is particularly crucial, as it needs to capture and understand the characteristics of different image domains and appropriately transform and reconstruct them. To enhance the realism of the images, the feedback from the discriminator is used to ensure the quality and accuracy of the generated image's style. Moreover, with the deepening of research, more variant models are proposed, such as UNIT and MUNIT, which introduce the concept of shared latent space, further enhancing the model's generalization ability and flexibility.
## 4.2 Applications of GANs in Audio and Text Processing
### 4.2.1 Speech Synthesis and Music Generation
In the field of audio processing, GANs have also opened up new application prospects, particularly in speech synthesis and music generation. GANs can generate natural and coherent speech signals and musical melodies. For example, by training GAN models, one can achieve a high degree of imitation of real human voices and generate new speech segments for application in text-to-speech (TTS) systems, significantly improving the quality of speech synthesis.
For music generation, GANs can learn from a vast number of musical works, capturing melody, rhythm, and style characteristics, and generate new, creative musical pieces. This shows great potential in music composition and personalized music recommendation systems. For example, the style of a particular musician can be integrated into the GAN model and then used to generate new works in the musician's style, aiding in the creative process.
### 4.2.2 Applications in Natural Language Processing
The field of natural language processing (NLP) also benefits from the development of GANs. In tasks such as text generation, machine translation, and semantic editing, GANs can enhance the performance of models by generating high-quality text samples. GANs can learn both the syntactic and stylistic features of text while maintaining correct semantics, generating text data that is realistic.
For example, in machine translation tasks, GANs can generate more natural and fluent translation results, improving translation quality. In text generation tasks, GANs can be used to generate text content with specific emotional tones or styles, such as news reporting or novel writing. Through continuous optimization of the generator and discriminator's adversarial process, GAN-generated text can be closer to the actual use of language in the real world.
## 4.3 Potential of GANs in Medicine and Science
### 4.3.1 Medical Image Analysis and Enhancement
Medical image analysis is one of the frontier areas of GAN applications. High-quality medical image datasets generated by GANs can improve the accuracy of lesion detection and diagnosis. For example, GANs can generate CT or MRI images containing specific lesions, assisting doctors in diagnosing diseases and planning treatments.
Additionally, GANs can be used to enhance medical images by improving the quality of low-dose scan images to that of high-dose scans. This method not only reduces the radiation dose for patients but also improves the image quality, thereby aiding more accurate diagnosis. For example, generative adversarial networks can enhance the quality of PET and CT images to compensate for image quality degradation due to equipment limitations or patient conditions.
### 4.3.2 Physical Simulation and Chemical Data Generation
In physics and chemistry research, GANs can simulate complex physical processes and chemical reactions. By learning experimental data, GAN-generated models can predict the structure and properties of molecules and simulate the physical and chemical characteristics of materials, which is valuable in drug discovery and new material development.
For example, in the field of cheminformatics, GANs can be used to generate the molecular structures of compounds, producing candidate molecules with specific properties. In physics, GANs can simulate the evolution of the universe, generating astrophysical data to help scientists better understand the mysteries of the cosmos.
In physical simulation applications, GANs need to accurately capture physical laws and chemical reaction kinetics to generate simulated data that is consistent with the real physical world. This usually requires incorporating physical law constraints into the GAN training process and ensuring the authenticity of the generated data through the discriminator.
## 4.3 Potential of GANs in Medicine and Science (Continued)
### 4.3.3 Bioinformatics and Genetic Data
Bioinformatics is a highly data-driven field where GANs are becoming an important tool, especially in the processing and analysis of genetic data. GANs can be used to generate new, potential genetic sequences, which has potential value in studying the function of genes, disease-related genetic variations, and developing personalized treatment plans. For example, by learning a vast amount of genomic data, GANs can generate genetic variations with specific disease-associated features, helping researchers better understand the mechanisms of diseases.
In generating genetic data, GANs need to ensure through the feedback of the discriminator that the generated sequences are both random and consistent with the biological laws of inheritance. This requires the generator not only to have strong data generation capabilities but also to understand the basic principles of bioinformatics.
Additionally, GAN applications in bioinformatics include simulating microbial communities, protein structure prediction, etc. In these tasks, GANs can provide a large number of reliable simulation data to supplement actual experimental data, thereby deepening the understanding of complex phenomena in life sciences.
## 4.3 Potential of GANs in Medicine and Science (Continued)
### 4.3.4 Climate Change and Environmental Science
In environmental science, GANs are changing our understanding of climate change and our ability to respond to it. By learning historical climate data, GANs can simulate future climate scenarios and predict the impact of climate change on the environment and socio-economic aspects. For example, GANs can generate climate model predictions under different emission scenarios for a specific region, providing a scientific basis for the formulation of climate policies.
The application of GANs in climate science is not limited to the generation of climate models but also extends to the enhancement of environmental monitoring data. For instance, in satellite remote sensing images, GANs can fill in missing data to improve the monitoring accuracy of land cover changes. This capability is particularly important for environmental protection and disaster assessment.
Additionally, the potential applications of GANs in environmental science include simulating natural disaster events such as floods, storms, and fires. By generating different scenarios of natural events, governments and organizations can develop more effective emergency response plans and mitigation strategies.
## 4.3 Potential of GANs in Medicine and Science (Continued)
### 4.3.5 Drug Discovery and Biomedical Engineering
In the fields of biomedical engineering and drug discovery, GANs offer new insights and tools. GAN-generated models can assist in designing new drug molecules that target specific diseases. This capability of GANs comes from the generator's creativity, which can produce a large number of novel and potentially effective compound structures.
In the drug discovery process, GANs can accelerate the screening and optimization of candidate drug molecules. It can learn the relationship between the structure and biological activity of compounds from known drug libraries and then generate a series of new drug molecules for experimental validation. At the same time, GANs can predict the biological activity of drug molecules, such as efficacy, toxicity, and metabolic stability, thereby reducing the number of experiments and costs in the drug discovery process.
Beyond applications in drug molecule design, GANs also play a role in the innovative development of biomaterials. For example, they can design new biocompatible materials for tissue engineering and regenerative medicine. By simulating the physical and chemical properties of various biomaterials, GANs help researchers predict the biocompatibility and functionality of materials, accelerating the development of new materials.
```mermaid
graph LR
A[GAN Research and Applications] -->|in Different Fields| B[Image Processing]
A --> C[Audio and Text Processing]
A --> D[Medicine and Science Field]
B -->|Face Recognition and Image Segmentation| B1
B -->|Image-to-Image Translation| B2
C -->|Speech Synthesis and Music Generation| C1
C -->|Natural Language Processing| C2
D -->|Medical Image Analysis and Enhancement| D1
D -->|Physical Simulation and Chemical Data Generation| D2
D -->|Bioinformatics and Genetic Data| D3
D -->|Climate Change and Environmental Science| D4
D -->|Drug Discovery and Biomedical Engineering| D5
```
Through the above analysis, GAN applications have penetrated into various levels of scientific research and industrial technology, and their potential is vast and diverse. With further research, we can anticipate that GANs will solve more complex problems in the future, driving the frontier development of science and technology.
# 5. Future Prospects and Challenges of GANs
## 5.1 Innovative Trends and Research Directions of GANs
### 5.1.1 Expansion and Improvement of Adversarial Learning
As the core of GANs, adversarial learning continuously drives the development of artificial intelligence. Innovative trends include the extension of adversarial learning to new fields and improvements in traditional problems. By creatively designing new network structures and loss functions, researchers continually challenge the limits of existing generation tasks. For example, the introduction of conditional adversarial networks (Conditional GAN, cGAN) can control the category or attributes of the generated content, thereby achieving new applications in image tagging, style transfer, and other fields. Additionally, the application of meta-learning methods in GANs is being explored, which can enable GANs to quickly adapt to new tasks and improve generalization capabilities.
```mermaid
graph LR
A[Start] --> B[Define the Problem]
B --> C[Choose the Appropriate GAN Model]
C --> D[Design the Loss Function]
D --> E[Conduct Model Training]
E --> F[Assess Model Performance]
F --> G[Model Optimization]
G --> H[Model Deployment and Application]
```
The code block shows a simple example of a cGAN model training:
```python
from keras.datasets import mnist
from keras.layers import Input, Dense, Reshape, Flatten, Dropout
from keras.layers import BatchNormalization, LeakyReLU, Conv2D, Conv2DTranspose
from keras.models import Sequential, Model
import numpy as np
# 1. Prepare the data
# ...
# 2. Build the generator model
def build_generator(z_dim):
model = Sequential()
# ... (Add network layers)
return model
# 3. Build the discriminator model
def build_discriminator(img_shape):
model = Sequential()
# ... (Add network layers)
return model
# ***pile the model
def build_gan(generator, discriminator):
model = Sequential()
# ... (Link the generator and discriminator)
return model
# 5. Train the model
# ...
# Model training logic explanation
# In this code block, we first prepared the dataset (not shown), then defined the generator and discriminator models. We combined the generator and discriminator to build the complete GAN model and compiled it. After that, in the model training step, we need to continuously train the GAN until we achieve satisfactory performance.
```
### 5.1.2 Integration of GAN with Other AI Technologies
GAN is not an isolated technology; it is currently integrating with other important AI technologies such as reinforcement learning and transfer learning, driving interdisciplinary development. In reinforcement learning, GANs can generate simulation environments for training robots, allowing them to learn complex tasks without real-world interaction. Additionally, GANs can be used for data augmentation to enhance the generalization ability of machine learning models.
The table shows typical application fields of the integration of GANs with other technologies:
| Application Field | Integrated Technology | Representative Achievement |
| --- | --- | --- |
| Image Recognition | Transfer Learning | Improve recognition accuracy on specific categories |
| Data Augmentation | Reinforcement Learning | Generate complex and varied training samples |
| Speech Synthesis | Temporal Prediction Models | Enhance speech quality and naturalness |
## 5.2 Ethical Issues and Solutions Facing GANs
### 5.2.1 Deepfakes and Regulation
With the rapid development of GAN technology, especially in the fields of images and videos, Deepfakes have become an increasingly concerning topic. Deepfakes use逼真 (highly realistic) videos or audio generated by GANs, which can be used to create false information, posing a serious threat to personal privacy and public safety. To address this challenge, the academic and industrial communities have begun to work together by developing technical means to detect and label Deepfakes, while calling for the formulation of stricter legal regulations to oversee the application of this technology.
### 5.2.2 Data Privacy and Model Transparency
When using GANs to process personal data, data privacy becomes an important consideration. Due to the powerful capabilities of GANs, they can use a small amount of data to generate逼真 (highly realistic) samples, which may lead to data abuse and privacy leakage issues. Therefore, researchers are committed to developing data protection mechanisms, such as differentially private GANs (Differentially Private GAN), ensuring that effective learning can still be carried out using GANs without disclosing personal information. At the same time, the transparency of models is also a current focus, ensuring that GAN-generated content can be identified and traced
0
0
相关推荐
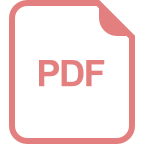
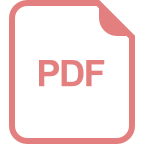
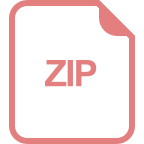
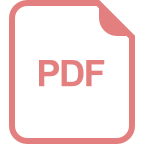
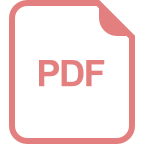
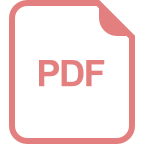
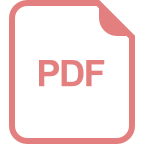
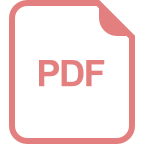
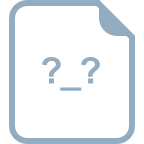